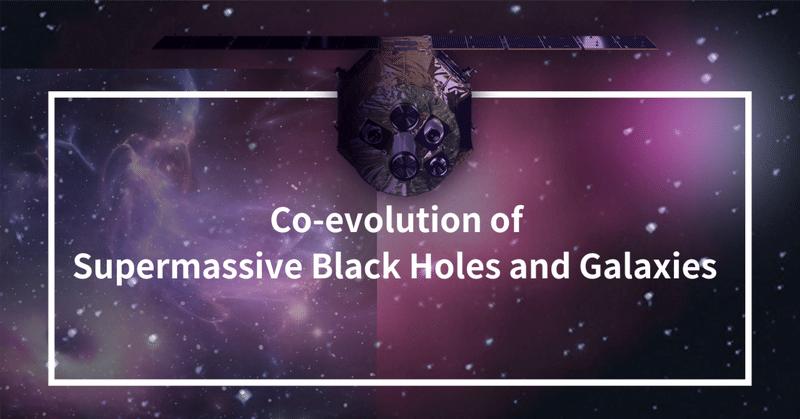
Co-evolution of Supermassive Black Holes and Galaxies Explored by XRISM
Galaxies are the fundamental elements of the universe, consisting of more than 100 billion stars. In the nearby universe, a SuperMassive Black Hole (SMBH) with a mass 100,000 to 10 billion times that of the Sun exists at the center of almost all galaxies, and its mass is found to correlate well with the total mass of stars in the galactic bulge (Figure 1 below). (Figure 1 below).
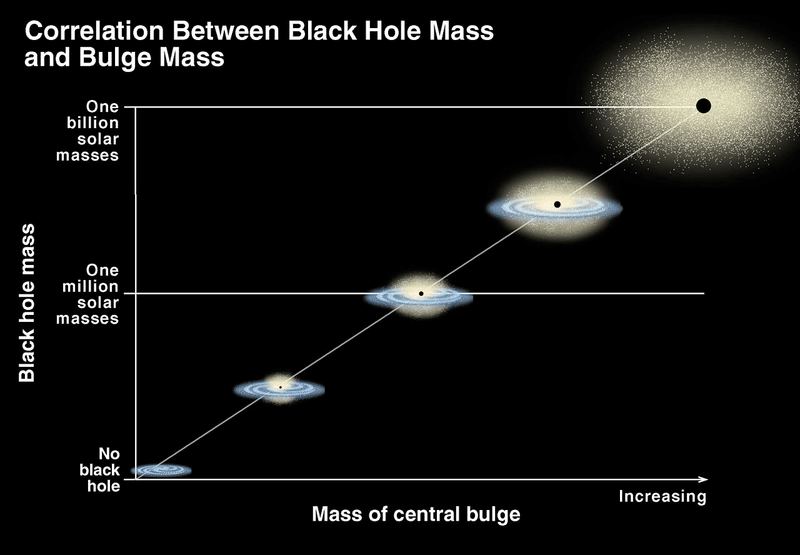
Credit: K. Cordes, S. Brown (STScI)
This observed fact strongly suggests that galaxies and black holes have evolved by closely influencing each other (co-evolution).
In other words, SMBHs, 10 orders of magnitude smaller than galaxies, are key players in the universe's evolution. The major remaining challenge for modern astronomy is to elucidate the mechanism of how galaxies and black holes co-evolved while influencing each other.
The Active Galactic Nuclei (AGN) are crucial for understanding co-evolution.
When the surrounding gas falls into the SMBH, it releases its enormous gravitational energy and becomes superheated. As a result, the galactic nucleus shines brightly in a wide range of wavelengths, from radio waves to gamma rays. At the same time, SMBH gains mass by inhaling gas. In other words, AGN is the very site where SMBHs grow.
Figure 2 shows a schematic diagram of an AGN.
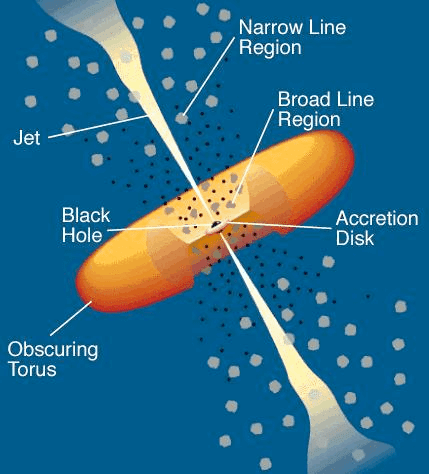
The accretion disk is thought to surround the central SMBH, surrounded by a torus of cold gas.
AGN Science with XRISM (1): AGN Feeding
A central mystery in the co-evolution problem is how the gas from the mother galaxy is transported to the SMBH. This is called AGN feeding, which is analogous to feeding the SMBH.
The AGN torus is located between the parent galaxy and the SMBH and is thought to be a reservoir of gas supplied to the SMBH, but its origin is not well understood. It is also believed that intense star formation occurs in the torus. Studying the torus's structure and the gas's motion inside it to determine its origin is essential to understanding the co-evolutionary process.
When X-rays emitted from the central nucleus enter a torus or other surrounding material and strike atoms or ions in it, X-rays (emission lines) of fixed energy are produced from them.
Figure 3 shows an XRISM observation simulation of AGN "Markarian 3" looking at the torus from the side.
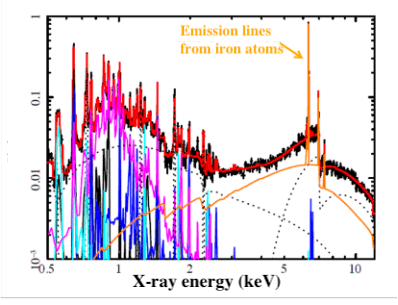
Ref. Reynolds et al. (2014).
The energy of emission lines emitted from a stationary material is fixed for each element. On the other hand, if the radiating object is moving, the observed energy is shifted due to the Doppler effect. Since light, like sound, has the property of a "wave," like the sound of a moving ambulance, the energy (frequency) appears higher when the emitting object is closer to the observer and lower when it is further away.
Using this principle, precise measurement of the energy of emission rays can reveal the movement of surrounding gases; unlike other wavelengths, diagnostics using X-rays allow us to see virtually any material over a wide temperature range.
The microcalorimeter with XRISM can study the motion of gases with 10 times greater precision than ever before, capturing the distribution of gases in the torus, their flow from the torus to the SMBH, and the return of some. This is expected to dramatically improve our understanding of AGN feeding.
AGN Science with XRISM (2): AGN Feedback
Another key to understanding co-evolution is a phenomenon known as AGN feedback.
AGNs not only emit strong electromagnetic radiation but also produce outflows such as jets and winds, which are thought to suppress star formation by warming and to push away the surrounding gas during the final stages of SMBH growth. This may result in a good correlation between the masses of galaxies and black holes.
However, many mysteries remain, including the physical origin of these outflows, the conditions under which they are produced, and whether they emit enough energy to affect the parent galaxy.
When a wind emitted from an AGN lies between the central continuous X-ray source and the observer, the ions in the wind absorb only X-rays of a particular energy. As a result, a "dent" structure (absorption lines) will be seen in the observed energy spectrum (energy distribution).
By measuring the deviation between the energy of the observed absorption lines and the original energy, the velocity of the wind can be determined by the Doppler effect, and the amount of material in the wind can be determined by measuring the depth of the "dent." From these measurements, we can also estimate the amount of energy the wind carries outward in a unit of time.
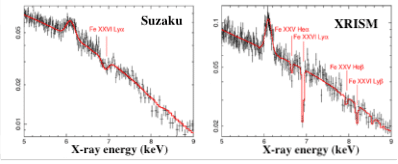
Ref. Kaastra et al. (2014).
The microcalorimeter onboard XRISM can observe absorption lines due to the AGN wind with unprecedented precision due to its superior spectroscopic capabilities (Figure 4).
In this way, we can expect to obtain the exact amount of energy outflow from the winds and to be able to settle on whether these are indeed affecting the parent galaxy.
Author: Yoshihiro Ueda
(This article was translated from Japanese.)