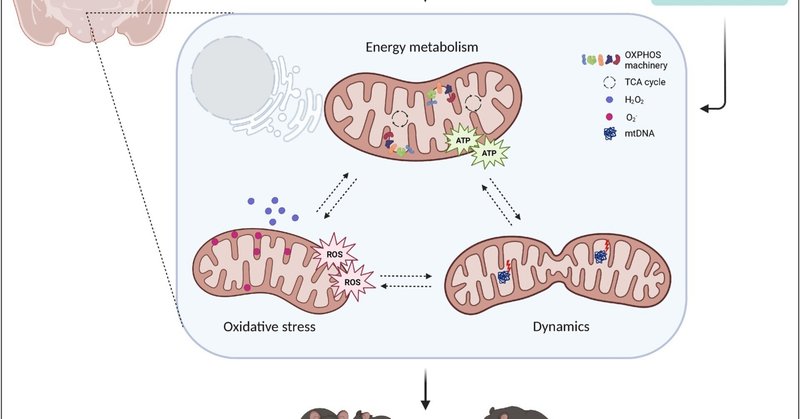
社会的な脳を動かす: 社会的行動におけるミトコンドリア
神経生物学のカレントオピニオン
第79巻、2023年4月、102675号
社会的な脳を動かす: 社会的行動におけるミトコンドリア
https://www.sciencedirect.com/science/article/pii/S0959438822001696
著者リンク オーバーレイパネルを開くDoğukan Hazar Ülgen a, Silvie Rosalie Ruigrok a, Carmen Sandi
もっと見る
概要
シェア
引用
https://doi.org/10.1016/j.conb.2022.102675Get 権利と内容
クリエイティブ・コモンズ・ライセンスに基づく
オープンアクセス
ハイライト
脳の特定部位におけるミトコンドリアの変異は、社交性や社会的地位の個人差と関連している。
ストレスによる社交性障害は、ミトコンドリアの機能障害と関連している。
ミトコンドリアの機能不全は、エネルギー代謝の変化、酸化的損傷、および/または脂質代謝の低下を含む。
ミトコンドリアを標的とした栄養補給は、社会的機能障害に取り組むための有望な介入戦略として浮上する。
概要
社会的行動の制御と影響における脳内ミトコンドリアの中心的役割が明らかになりつつある。ミトコンドリアは、細胞の「発電所」としての重要な役割に加え、イオン恒常性、神経伝達物質レベル、脂質代謝の調節など、多くの細胞機能を有している。ここ10年の研究で、社会的な領域を含む行動の制御において、ミトコンドリアが不可欠な役割を担っていることが明らかになりつつある。ここでは、ミトコンドリアの機能と動態を社会的行動や障害に関連付ける最近の証拠について、社会的行動がミトコンドリアの変化という文脈でストレスによって調節される例を含め、治療戦略の可能性やこの分野における未解決の疑問について述べる。
前の記事
次の記事
はじめに
動物界では、ヒトを含む多くの種が社会性を持つように進化してきた。なぜなら、捕食者の多い環境で一人でいることは、多くの危険をもたらすからである。そのため、私たちの脳は、他の多くの種の脳と同様に、広範囲に渡って社会的である。このことは、視床下部核、側坐核(NAc)、腹側被蓋野(VTA)、扁桃体、海馬(HIP)、前頭前野(PFC)など、社会的手がかりの処理と統合に関わるものから、報酬、動機、意思決定、行動制御に関わるものに至るまで、さまざまな脳領域と社会的領域に関わる相互接続回路の多さに反映されています [1,2]. 社会的行動の変化は、うつ病、不安障害、自閉症など、ほとんどの精神疾患で見られます [3, 4, 5]。これらの疾患では、社会性の低下や、社会集団に溶け込めない、ペアボンドを形成できない、他者に対して攻撃的になるなどの問題がしばしば見られます。慢性的なストレスは、異常な社会的行動と精神疾患の両方を発症させる重要な危険因子である [1,6]。したがって、社会的機能と機能不全を支配する神経メカニズムを理解することは、最も一般的な精神疾患のいくつかの背景を見極めるための重要なステップであり、治療介入のための新しい道を明らかにするかもしれない。
最近の新たな証拠により、脳のミトコンドリアは、社会的変化を伴う精神病理を含む脳の(機能)障害にスポットライトが当てられています [7,8]。ミトコンドリアは、細胞のエネルギー代謝、イオン恒常性、脂質代謝、シグナル伝達などに重要な役割を果たす二重膜の小器官です(囲み記事1参照)。本総説では、ミトコンドリアの機能とダイナミクスを社会的行動に関連付ける最近の知見に焦点を当て、ミトコンドリアの変化と関連するストレスによって社会的行動が変化する例とともに、治療戦略の可能性と将来の展望を議論します(図1)。
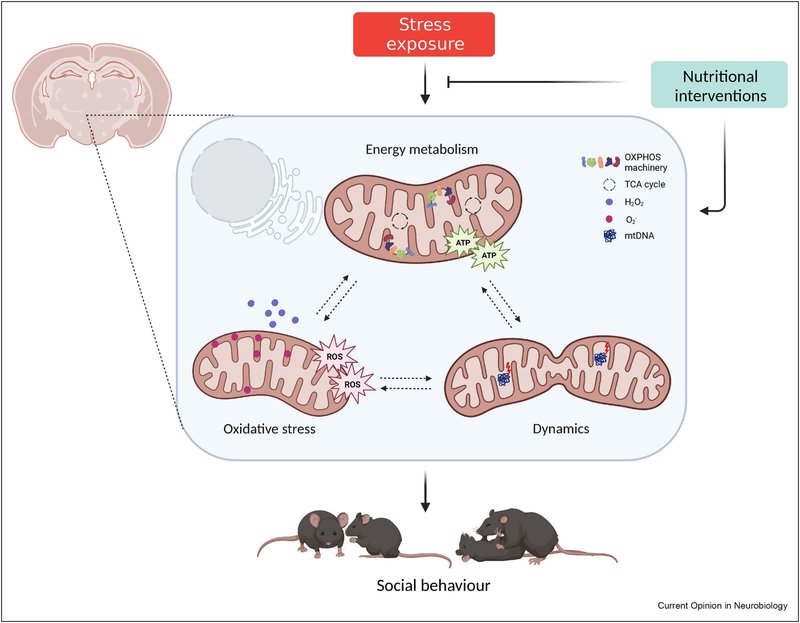
ボックス1
ミトコンドリアの機能と動態
ミトコンドリアは、生体エネルギーと「シグナル伝達器官」の両方を兼ね備えている [68] 。ミトコンドリアの内膜は、主に酸化的リン酸化(OXPHOS)によるアデノシン三リン酸(ATP)の生産に関与している。外膜は、リソソームや小胞体などの他の小器官との膜接触部位を形成し、ミトコンドリアのシグナル伝達の主要なプラットフォームであるとともに、ミトコンドリアの分裂(分割)と融合に不可欠である[69]。ミトコンドリアは、大きくなるために融合を行い、例えば、エネルギー需要が高いときにそうする。分裂は、ミトコンドリアが損傷したときにしばしば起こり、マイトファジー(ミトコンドリアの分解)の対象となるはずである。さらに、ミトコンドリアはステロイドホルモンを合成し、アポトーシスを制御し、細胞内のイオン恒常性を調節する(文献[69,70]にレビューあり)。ミトコンドリアの形態は、その機能に強く関係している[71]。融合/分裂のダイナミクス、全体的な形態(長さ、幅、丸み)、超微細構造(内膜と外膜)のいずれも、異なる細胞種、細胞周期段階、代謝シグナルに応答して変化する。
ほとんどの細胞種は、ATPの生産をミトコンドリアのOXPHOSに大きく依存しているが(例えば、ニューロン)、例外もある(例えば、解糖に依存するアストロサイト)[72]。OXPHOSが行われる前に、グルコース、脂肪酸、アミノ酸が異化される必要がある。得られた生成物はミトコンドリア内のトリカルボン酸(TCA)サイクルに入り、ミトコンドリア内膜にある電子輸送鎖(ETC)によってOXPHOSに使用される基質を生成する [73] 。ETCは4つのタンパク質複合体(複合体I~IV)から構成され、電子が移動することでプロトン勾配(ミトコンドリア膜電位とも呼ばれる)が形成されます。OXPHOSプロセスは、TCA由来のNADHをNAD+に還元して生成した電子の放出から始まり、ATP合成酵素(複合体V)によってアデノシン二リン酸(ADP)にリン酸が付加されてATPが生成されて終了します。OXPHOSの際、酸素は電子受容体として働き、そのためミトコンドリアの酸素消費量はミトコンドリア活性の代理として使用することができる。
活性酸素種(ROS)は、電子の漏洩によりOXPHOS中の自然な副産物として生成され、酸素のO2--への還元を引き起こし、通常スーパーオキシドディスムターゼ(SOD)によってH2O2に急速に変換される(文献[74]で詳しくレビューしている)。H2O2はミトコンドリアを離れ、シグナル伝達分子として機能し、細胞増殖や分化などのプロセスを制御することができる。生理的な役割にもかかわらず、高レベルの活性酸素は、膜脂質やDNAの酸化により、細胞にダメージを与える可能性があります。そのため、グルタチオン(GSH)系などの抗酸化システムが設定され、高レベルの活性酸素から保護し、その後、細胞やミトコンドリアの損傷を防いでいます。
ダウンロード 高解像度画像のダウンロード (569KB)
ダウンロード フルサイズ画像のダウンロード
図1. ミトコンドリアの機能と動態は、社会的行動とストレスによる社会的欠損の中心である。ミトコンドリアを標的とした栄養学的介入は、社会性と社会的地位を調節する。ATP、アデノシン三リン酸、ROS、活性酸素種、OXPHOS、酸化的リン酸化、TCA、トリカルボン酸、mtDNA、ミトコンドリアDNA。
ミトコンドリアと社会的競争
社会階層の形成は、労働や資源の分担など、集団の恒常性を維持するために極めて重要であり、社会的競争において支配的または従属的になる確率は、-ある程度-生物学的に決定されている [10] 。特定の脳領域におけるミトコンドリア機能が社会的行動に重要であることを示す最初の証拠は、社会的競争に関連するものでした。意欲的な行動の脳内ハブである側坐核(NAc)は、競争にさらされると非常に活性化し、その抑制によって社会的優位性が低下するため、競争的な出会いの結果に決定的に関与することが示されていました[11,12]。2015年、Hollisら[12]は、NAcのミトコンドリアが社会的競争において重要な役割を果たすことを明らかにしました。彼らは、特性や生活史は同じだが不安気質が異なる2匹の雄ラットが新しい縄張りを奪い合ったとき、高不安(HA)ラットは低不安(LA)相手よりも従属的になりやすいことを示しました。驚くべきことに、これらの行動結果は、NAcの神経細胞やミトコンドリアの構造や機能の変化と関連していた。LAと比較して、HAラットは中棘ニューロンの樹状突起の萎縮と成熟スパインの密度の減少を示し、興奮性入力の頻度も減少した [13] 。これらの形態的な違いは、ミトコンドリア関連遺伝子の発現の増加を伴い、これらの知見と一致するように、HA動物のNAcではLAと比較して、ミトコンドリア複合体IおよびIIタンパク質レベル(ボックス1参照)、呼吸能力およびATPレベルが低く、ROS生産が高いことがわかった [12,13]. 重要なことは、NAcにニコチンアミドを注入してミトコンドリア機能を高めると、HAラットの劣等感傾向が消失したことである [12]。NAcニューロンのミトコンドリア密度はどちらの不安グループでも同様であったが、その後の研究でミトコンドリアの形態が大きく異なっていることがわかった。HAラットのミトコンドリアはLA動物に比べて球状で量が多く、小胞体(ER)との接触の数が非常に少なかった[13]。したがって、ミトコンドリアの動態や小胞体との相互作用に関わるタンパク質が、観察された表現型に関与している可能性がある。さらなる研究により、NAcのミトフシン2(MFN2;ミトコンドリアの融合とミトコンドリアとERの相互作用を制御するタンパク質)のレベルの低下が、HAラットのミトコンドリア、神経細胞および行動の表現型に因果関係を持つことが明らかになった[13]。これらの知見を総合すると、脳の構造や機能、意欲的な行動における個人差を説明する上で、NAcにおけるMfn2発現の自然変異が重要な役割を果たすことが明らかになった[14]。
この確立された不安と社会的競争のラットモデルは、社会的競争とNAcのミトコンドリア機能の両方が、抗不安薬ジアゼパムの投与によって調節されることも明らかにした。HAラットにジアゼパムを末梢(低用量)または腹側被蓋野(VTA)(NAcに投射するドーパミン作動性ニューロンを含む脳領域)に投与すると、LAラットに対する競争上の不利が回復した [15]. この効果は、ドーパミン受容体1(DRD1)の活性化により、ミトコンドリア機能(呼吸とATP産生)を一時的に高めるNAcへのドーパミン放出の増加に関与することが示されたことは重要である。NAcの複合体I関連ミトコンドリア呼吸を阻害すると、ジアゼパムの効果がなくなり、最終的にNAcのミトコンドリア機能とドーパミンシグナルが関連づけられた[15]。これらの知見は、NAc DRD1シグナルを介して社会的行動を制御するVTA-NAc投射を示す以前の文献と一致しており[16]、この関連性におけるミトコンドリアの重要な役割を暗示することによって、これらをさらに拡大するものである。
雄ラットにおいて社会的競争力を制御する脳のミトコンドリア機能を特定したのに続き、社会的地位もまた、末梢組織におけるいくつかの種でミトコンドリア機能に関連している。例えば、従属的なマウスは脾臓で酸化的リン酸化に関わる遺伝子の高い発現を示し、支配的なマウスは肝臓でカルボン酸や脂質の異化過程に関わる遺伝子の高い発現を示した [17]. シクリッド魚では、安定した(しかし不安定ではない)階層において、支配的なオスは部下と比較して血漿中の活性酸素種(ROS)レベルの増加を示した [18].また、肝臓の遺伝子発現解析では、従属的なニジマスではミトコンドリアの分裂が多く、融合が少ないことが示されました [19]。アカゲザルの雌では、グルーミングに費やした時間(上位の地位を示す所属行動の指標)と、B細胞におけるmtDNAコピー数は正の相関がありました(攻撃的行動による順位ではなく)[20]。これらの研究を総合すると、異なる種や異なる組織・細胞タイプにおいて、ミトコンドリア機能が社会的地位に依存して変化していることがわかります。
ミトコンドリアと社会性
その後の研究により、ミトコンドリアは社会的地位の確立における役割を超えて、社会的行動の他の側面にも関与していることが示されました。例えば、mtDNA遺伝子Nd6の変異によるミトコンドリアの欠損は、雄マウスの社会的選好課題における社会性の低下と関連している [21] 。興味深いことに、社会的一夫一婦制のプレーリーハタネズミでは、ペアボンドリングは、雌ではなく雄のNAcにおけるmtDNAコピー数の増加を伴い、ミトコンドリア分裂を制御する遺伝子(Fis1、Dnm1l)の発現における性特異的変化、同居後の雄のNAcにおける分裂増加を示しています[22]。
無脊椎動物における最近の研究により、ミトコンドリアが社会的行動を制御する基本的なメカニズムについて、さらなる洞察が得られている。具体的には、自閉症や統合失調症に関連する細胞骨格ダイナミクスやタンパク質翻訳を制御するタンパク質であるヒトCYFIP1のホモログに変異を持つショウジョウバエは、社会集団行動における障害(例えば、社会集団内のハエ間の距離増加や求愛行動の減少)を示し、脳におけるミトコンドリア呼吸、ミトコンドリアサイズの増加、TCAサイクル活性上昇を伴っていた [23]. 変異体のミトコンドリアにはGABAが蓄積されていたことから、変異体の社会性障害はGABAシグナルの欠陥と因果関係があることがわかった[23]。GABAの取り込みは、ミトコンドリア外膜に存在する溶質輸送体Aralarによって推進され、アスパラギン酸をグルタミン酸とプロトンに交換することが以前に報告されている[24]。社会的行動の調節におけるGABA作動性シグナルの関与と並んで、アストロサイトにおけるエネルギー代謝もまた、雄マウスの社会的行動に影響を与えることが示されている[25]。実際、社会的選好課題におけるカンナビノイド誘発性の社会的欠損は、アストロサイトのミトコンドリアまで遡ることができた。ミトコンドリア膜に関連するアストログリアの1型カンナビノイド受容体(mtCB1)を活性化すると、ミトコンドリア複合体Iの安定性と活性が低下し、アストロサイトのROS生産が低下した。アストロサイトの活性酸素が低下すると、アストロサイトでの乳酸産生が妨げられ、神経細胞で酸化還元ストレスが発生する。その結果、神経細胞で過剰に生成された活性酸素が、カンナビノイドによって誘発される社会的相互作用の障害を媒介した。このように、ミトコンドリアは、ニューロン内のGABAを隔離することによって直接的に社会的行動を制御することができるが、アストロサイトからニューロンへの代謝支援を低下させることによっても間接的に社会的行動を制御する。
不安障害、うつ病、自閉症などの精神障害に見られる社交性の低下とは対照的に、社交性の低下はウィリアムズ症候群(WS)の顕著な特徴である [26] 。WSは7番染色体(7q11.23)から26-28個の遺伝子が欠失することで発症し、この領域の重複は自閉症と関連している[27]。注目すべきは、ミトコンドリア機能もWSの病因やそれに関連した社交性の向上に関連していることです[28]。WS患者の線維芽細胞は、ミトコンドリア呼吸とATP合成の低下と活性酸素産生の増加を示し、WS患者の大脳新皮質におけるOXPHOS複合体タンパク質レベルの変化と一致している。DNAJC30ノックアウトマウスでは、神経細胞の形態やミトコンドリアの変化とともに、社会的関心が高まっていることから、ATP合成機構の補助的な構成要素である7q11.23タンパク質DNAJC30が、これらのミトコンドリアの変化を一部促進することが明らかにされた。
このように、ミトコンドリア機能の亢進と低下はともに社会性の障害と関連しており [23,25]、ミトコンドリア機能の低下は社会性の低下とも関連している [28] 。したがって、ミトコンドリア機能と社会的行動の関係は直線的ではなく、むしろ活動が少なすぎたり多すぎたりすると異常が生じる放物線上にあるようです。
ヒトの場合、脳のミトコンドリアは死後しかアクセスできないため、社会的行動と脳のミトコンドリア機能の関係を研究することは困難である。1H-磁気共鳴分光法で測定された脳内代謝物は、代わりに生きている個人のミトコンドリア機能やその他の代謝プロセスの代理人として機能します。そのため、健常人を対象とした最近の研究では、前帯状皮質背部のN-アセチルアスパラギン酸(NAA:神経細胞のミトコンドリアで生成されるTCAサイクルの主要基質であるアセチルコエンザイムAの貯蔵型 [29])レベルと社会性、攻撃性などいくつかの感情構成との間に関連が示されました [30]. これらのデータは、齧歯類で上述した脳のエネルギー代謝と社会的行動の間のリンクが、ヒトにも存在することを示唆している。しかし、この研究はサンプルサイズがかなり小さいため、注意が必要である。重要なことは、遺伝子変異や薬理学的操作によってミトコンドリア機能が損なわれた動物における知見[12,13,15,21,23]と同様に、ミトコンドリア病のヒト患者もしばしば精神症状を示すことである(文献[31]にレビュー)。また、逆に、自閉症スペクトラム障害のような社会的行動の変化を伴う精神病理学的状態(文献[32]を参照)や、うつ病(文献[33]を参照)においても、(脳)エネルギー代謝の変化が確認されている。しかし、脳のエネルギー代謝の変動が、ヒトの非病理的な条件下での社会的行動にも関係しているかどうかについては、さらなる調査が必要である。
ストレスによる社交性の低下はミトコンドリアに起因する
個人の生涯を通じて様々な時期に発生するストレスは、ヒトだけでなくげっ歯類においても、社会的行動に強い影響を与えることがあります[1]。気分や意欲、不安の変化とともに、身体的および心理社会的ストレスは、社会性の低下や攻撃性の上昇につながる可能性があります [1,34]。現在、脳内のミトコンドリアの変化が、ストレスの影響とその結果としての社交性障害に関与していると考えられている。ここでは、幼少期と成人期のストレスに関するエビデンスをレビューする。
早期ストレスによる社会的行動のプログラム-ミトコンドリアとの関連性
早期のストレス体験は社会的行動に強い影響を及ぼし、社会的動機の低下、反社会的特徴の発現、成人後の攻撃性の上昇につながることが多い(文献[1]にレビューあり)。また、ストレス体験は、PFC、HIP、視床下部など、社会的行動の制御に重要な役割を果たすいくつかの脳領域の構造と機能に影響を与える可能性があり、これらの知見はヒトとネズミの両方について報告されています。
さらに、早期のストレス体験への曝露は、脳のミトコンドリア機能をプログラムする可能性があり [35, 36∗∗, 37, 38, 39] 、現代の知見は、これらのミトコンドリアの変化をストレスによる社会行動の変化に結びつけています。まず、雄ネズミの思春期前後のストレスが攻撃性の増加[35]や後年の社会性障害[36]につながり、それぞれ扁桃体(呼吸増加)[35]やNAc(呼吸減少)[36]のミトコンドリア機能異常と関連しているという相関証拠が研究報告されている。第二に、最近の研究では、末梢と脳のエネルギー代謝の両方が、思春期前後のストレスによる社会的欠損と因果関係があるとされています[36]。具体的には、思春期前後にストレスを受けた雄マウス(雌は含まない)は、成人期に社会性の障害と脂肪率の上昇を同時に示すことが判明した。驚くべきことに、脂肪中のアディポカインであるニコチンアミドホスホリボシルトランスフェラーゼ(NAMPT)および血中の細胞外型eNAMPTのレベルが低下していることが、社交性の低下に寄与していることが判明した。eNAMPTの減少は、NAcのニコチンアミド・アデニン・ジヌクレオチド(NAD+)レベルの低下、ひいてはSIRT1経路の活性の低下を招いたと考えられている。これらの変化は、NAcの中棘ニューロンの発火頻度の低下とそれに伴う社交性の変化につながった。重要なことは、思春期前後のストレスにさらされた後、NAD+ブースターであるニコチンアミドモノヌクレオチド(NMN)で処理すると、成人後の社会性障害の発現が抑制されたことである[36]。思春期前後のストレス以外にも、出生前や出生後早期のストレスは、ミトコンドリア低酸素マーカーである2-ヒドロキシグルタル酸のレベル上昇とともに、成人後の社会的欠損や抑うつ様行動をも引き起こす [37].さらに、ミトコンドリア活性化化合物であるアセチル-L-カルニチン(ALC)の介入は、マウスをうつ病様行動の発症から保護しました[37]。これらの研究を総合すると、幼少期の経験が、少なくとも部分的にはミトコンドリアの変化を通じて、その後の社会的行動をプログラムすることができることがわかります。
成人期のストレスによる社会的行動の変化-ミトコンドリアとの関連性
成人期にストレス要因にさらされると、社会的行動にも影響を及ぼし、しばしば社会的回避や未知の同属動物に対する恐怖につながります [1] 。成人期のストレスによる社交性障害にミトコンドリアが関与しているとする研究の多くは、社会的敗北パラダイムを利用しています。雄マウスでは、社会的敗北によって扁桃体基底核と中心核のミトコンドリアサイズと質量が減少することが示された [40] 。それに伴い、ATPを得るために利用されるミトコンドリアの膜電位[41]も、これらの脳領域で低下していることが判明した。ストレスに弱い動物では、mtDNA変異の増加とmtDNAコピー数の減少が見られ、マイトファジーのレベルの上昇と関連していることが分かりました。マイトファジーをダウンレギュレートすると、不安様行動が消失した。さらに、ストレスに弱い雄マウスの海馬ニューロンのミトコンドリアも、マイトファジーとオートファジーの亢進によって悪影響を受け、神経細胞の成長と発達が阻害されることが判明した[42]。神経細胞の正常な機能には、無傷で機能的なミトコンドリアが不可欠であり、マイトファジーとオートファジーは、ミトコンドリアの「質」を厳しく監視している(ボックス1)。最適でない構造や変異したmtDNAによって機能が低下した神経細胞ミトコンドリアは、特にマイトファジーによって標的化できない場合、最終的にストレス様表現型に寄与するさまざまなメカニズム(例えば、細胞エネルギー欠損、カルシウム緩衝の変化、神経伝達物質生成、その他)を通して神経回路の機能に影響を与える可能性がある。
他の研究では、社会的敗北ストレスによって複数の回路が影響を受ける可能性があることが示されている。例えば、雄のC57BL/6マウスの線条体終末床核(ストレス反応に関連する別の領域)から得られた統合マルチオミクス解析 [43] では、社会的敗北ストレスによって酸化的リン酸化が上昇し、一方で別のミトコンドリア関連経路、SIRT1シグナル伝達が低下していることが明らかになりました [44] 。興味深いことに、ストレスを受けたDBA/2雄マウスでも、同じ経路が社会的敗北によって影響を受けることがわかったが、その方向は逆であり、遺伝子制御の系統間差が強調された。これらの結果から、ミトコンドリアのホメオスタシスは社会性脳にとって極めて重要であり、この絶妙なバランスが崩れると、ストレスによる社会性の欠陥につながる、あるいは影響されることが明らかになった。
ミトコンドリアのホメオスタシスは、細胞内の活性酸素濃度の調節に決定的に関与している。活性酸素は酸化的リン酸化の副産物として発生し、一定レベルは細胞機能に有益であるが、高レベルではDNA、タンパク質、脂質に損傷を与える可能性がある(Box 1を参照)。社会的敗北は、HIPにおけるグルタチオン還元酵素1、グリオキサラーゼ1、スーパーオキシドジスムターゼ(SOD)などの酸化ストレス酵素のタンパク質レベルの減少につながることが示されている[45]。さらに、ストレス耐性動物はNAcと腹内側PFCにおいてシスチン(抗酸化物質GSHに変換されるシステインの二量体)の濃度が上昇していることが判明した[46]。攻撃的なマウスのPFCでは、酸素消費率やATP合成量に差は見られなかったが、シトクロムcオキシダーゼのレベルや活性は低いことがわかった[47]。この調節障害は脳にとどまらず、社会的に孤立したラットでは、GSHの減少とともに、肝臓でも活性酸素とSOD活性が増加した[48]。ストレスによって生じる神経細胞の興奮の変化が活性酸素の産生を増加させることに加えて、ストレスはストレスホルモンの転写作用を通じて酸化に影響を与える可能性があります。特に、細胞の酸化ストレスに比例してmRNAとタンパク質レベルが変化するトランスサイレチン遺伝子は、その第1イントロンにグルココルチコイド受容体応答要素を含み、ストレスによって発現が上昇することが分かっています(文献[49]にレビュー)。これらのことから、ミトコンドリア機能不全による活性酸素の増加が、ストレスの根本的なメカニズムに関与していることが示唆されます。
ミトコンドリア機能不全は、通常、エネルギー代謝や脂質代謝の変化を伴う、広範な原因と影響を及ぼす可能性があります。実際、多くのプロテオミクス、リピドミクス、代謝に関する研究が、ストレスによる潜在的な障害を示唆している。雄ラットでは、社会的隔離により、HIPから得たシナプトソーム画分中のグルコース代謝と小胞輸送タンパク質が増加する [50] 。一方、細胞質画分では、解糖系酵素から酸化的リン酸化へのシフト、ミトコンドリア関連の酸化還元系とカルシウムシグナルのダウンレギュレーションが観察されている[51]。雄マウスでは、社会的孤立がPFCの脂質過酸化を誘発し、エネルギー需要の増加を示唆している[52]。社会的敗北ストレスを受けた雄ラットの扁桃体とPFCでは、NAAが増加することがわかった [53]。一方、社会的敗北ストレスにさらされた雄ラットのHIPでは、乳酸とNAAの両方が増加し、それぞれ解糖とミトコンドリア機能不全の増加を示唆した[54]。社会的敗北ストレス感受性マウスの内側PFCではグリコーゲンの蓄積が観察され、アストロサイト脳型グリコーゲンホスホリラーゼPYGBの機能不全は、グリコーゲン蓄積と社会的敗北ストレス時に観察されるうつ様行動を誘発するのに十分であった[55]。慢性的な社会的敗北ストレスは、腹側HIP、NAc、mPFCにおいて、代謝物や脂質の強く多様な変化をもたらした[56]。変化する代謝物は、プリンヌクレオチド、カルニチン供与体、β酸化に関与する修飾脂肪酸、抗酸化物質に及んだ。最後に、LACを補充したマウスは、高位にあるマウスの慢性拘束ストレスの悪影響を生き延びた[57]。LACは長鎖脂肪酸のミトコンドリアへの輸送に関与しているので、この輸送を促進することは、エネルギー代謝を脂質酸化に有利に傾け、それによってストレスの解糖促進エネルギー代謝に対抗する可能性が高い[58]。これらの知見を総合すると、エネルギーおよび脂質代謝の欠陥は、ミトコンドリア機能障害への道を開くか、あるいはミトコンドリア機能障害に由来する可能性があり、脳における社会的ストレスの影響を先導する可能性があることが強調される。
栄養学的介入戦略
ミトコンドリアのエネルギー代謝が社会的行動を制御しているという事実(文献[12,36]など)、および食事と特定の栄養素が脳のミトコンドリア機能に影響を与えるという事実 [59,60]から、社会的行動を食事の補充によって調節することができるかという疑問が出てきた。すでにいくつかの新しい研究が、この見解を支持している。例えば、思春期前後のストレスによって誘発された社会的障害は、NAD+ブースターであるニコチンアミドモノヌクレオチドの食事による補給によって回復することができた [36] 。別の研究では、中鎖トリグリセリド(ミトコンドリアの効果的なエネルギー源)を補給することで、不安の強いラットの社会性が向上することが示されました [61] 。NAcではなくmPFC内のミトコンドリア機能の向上が、これらの食餌効果に関連していた。さらに、ミトコンドリア増強剤であるLACの補給は、慢性的なストレスにさらされた高階級-低階級ではない-マウスにおいて有益な効果を発揮した[57]。実際、高ランクのマウスを慢性ストレスに暴露すると、うつ病様行動が増加し、ホスホクレアチン(エネルギー代謝に関与)やタウリン(抗酸化物質)などの代謝物のレベルが低下したが、LACの補給により、これらの代謝物のレベルはコントロールレベルまで逆転した。最後に、セロリ(Apium graveolens)の種子から分離されたl-3-n-ブチルフタリドに基づく合成化合物であるDl-3-n-ブチルフタリド(NBP)の胃内投与は、ストレス誘発性の社会的欠損、不安様行動および絶望、ならびに解糖およびTCAサイクルの代謝物レベルの変化を軽減させました[62]。したがって、栄養学に基づく介入は、(ストレスによって誘発される)社会的障害の潜在的な治療ターゲットとなる。
結論と今後の展望
ミトコンドリアは、社会的行動の制御における重要なオルガネラとして浮上しており、それゆえ、ミトコンドリア機能の変化がストレスによる社会的行動の変調に関与していることもここで証明した。脳ミトコンドリアの問題は、エネルギー代謝のアンバランス(解糖と酸化的リン酸化)、イオンホメオスタシスの乱れ、細胞内の活性酸素レベルの上昇、脂質代謝の低下(β酸化または過酸化)など、様々な形で現れる可能性がある。介入戦略としては、NAD+ブースター、NBPまたは中鎖脂肪酸の補給が考えられる。
多くの未解決の問題がある。前臨床研究のほとんどは、男性のみを対象としています。男女を含む研究はほんの一握りで、男女間のミトコンドリア機能の違いはすでに報告されている。例えば、社会的孤立ストレス時の活性酸素に対する感受性は、雄マウスよりも雌マウスの方が高く[63]、mtDNAコピー数はペアボンドをした雄でのみ増加し、雌のプレーリーハタネズミでは増加しない[22]。したがって、同様の脳回路、細胞および分子プロセスが雌の社会的行動に関与しているかどうかについては、さらなる調査が必要である。
社会的行動は、末梢やホルモンの調節だけでなく、関与する多くの脳領域のレベルでも相互に関連しているため、行動反応のこれらの異なる側面におけるミトコンドリアの役割を明らかにすることは、これまでのところ困難であった。例えば、ドーパミンは社会的行動を調節することが知られており、NAcのドーパミンシグナルが増加するとミトコンドリア機能が高まり、行動出力に変化が生じることが示されている[15]。この研究をオキシトシンなどの他の行動制御ホルモンにも広げ、ホルモンと行動の間のどのリンクがミトコンドリアの基盤になっているかを理解することが不可欠であろう[64]。
このレビューを通して概説したように、ミトコンドリアの機能とダイナミクスは、社会的行動の多くの異なる側面や、不安やストレスに関連した行動の変化に関与してきた。しかし、ミトコンドリアの変化は、i)社会的行動の変化の主要な原動力であるか、ii)変異した転写因子による転写調節障害、クロマチントポロジーの基本的な違い、循環因子など、別の基礎となる(神経)生物学的差異に対する二次効果である可能性のどちらかです。ミトコンドリアが行動を制御する主要な基質であることを示す証拠として、脳室内ミトコンドリア移植がマウスとラットにおいてそれぞれ不安と抑うつ様行動を改善したことを示す最近の研究がある [65,66] 。また、別の研究では、ミトコンドリア内膜に位置し、ミトコンドリアによるCa2+の取り込みを調節するBcl-2タンパク質の変異が、マウスがより不安様行動を示すようになったことが示されている[67]。一方、血中のアディポカインであるNAMPTの量が減少すると、思春期ストレスモデルにおける行動異常やNAcのミトコンドリア欠損の原因となることが示され、NAMPT量を補充することでこれらの影響が解消された [36]。ミトコンドリアが細胞機能、ひいては脳機能の中心であることを考えると、ここで紹介した両方の見解が真実であると考えられる。ミトコンドリアの変化は、ある行動異常の原動力であるかもしれないが、他の神経生物学的差異のために二次的である可能性もある。特定のミトコンドリア異常(ミトコンドリア遺伝子の変異)を特定の行動障害と関連付け、その異常をモデル化してトランスジェニック動物モデルで障害を再現することで、この議論に光を当てることができるようになるでしょう。
もう一つの重要な問題は、社会的行動を支配するミトコンドリア機能に、細胞タイプに特異的な違いがあるかどうかを理解することである。このような細胞型特異性を報告した研究はいくつかあるが [25,55]、異なる細胞型における社会行動へのミトコンドリアの特異的寄与を明らかにするためには、さらなる研究が不可欠である。最後に、ミトコンドリアの代謝機能と基質への依存性、およびその生成物は、栄養学的または薬学的なターゲティングに適していることから、この分野の新たな知見は、ミトコンドリアを標的としたアプローチの治療可能性を調査する上で有望である。
利益相反声明
特になし。
謝辞(Acknowledgements
本研究は、スイス国立科学財団(SNSF)[176206および197942、NCCRシナプシー:51NF40-158776および-185897]、ERA-NET(バイオストレス:SNSF番号31NE30_189061)およびEPFLからの学内資金によりC.Sに助成されました。SRはSNSFスイス博士研究員の資金(助成番号TMPFP3_210670)より提供されています。
特集記事おすすめ記事
データの利用について
論文に記載された研究に使用されたデータはありません。
参考文献
1
C. サンディ、J.ハラー
ストレスと社会的脳:行動学的効果と神経生物学的メカニズム
Nat Rev Neurosci, 16 (2015)
グーグル スカラー
2
S.D. Lanooij, U.L.M. Eisel, W.H.I.M. Drinkenburg, E.A. van der Zee, M.J.H. Kas
社会的相互作用を介して認知パフォーマンスに影響を与える:神経解剖学的マッピングに基づく脳障害の新しい治療アプローチ?
Mol Psychiatr (2022), 10.1038/s41380-022-01698-1
グーグル・スカラー
3
I.M.J. サリス、M. アガジャニ、S.J.A. ファンデルヴェルフ、N.J.A. ファンデルウィー、B.W.J.H. ペンニックス
うつ病と不安障害のある患者における社会的機能
Acta Psychiatr Scand, 136 (2017), pp.352-361
CrossRefScopusGoogle Scholarで表示する。
4
U. フリス、F.ハッペ
自閉症スペクトラム障害
Curr Biol, 15 (2005), pp.R786-R790
PDFを見る記事を見るScopusGoogle Scholarで見る
5
F. シュラグ、A.G.アレグリーニ、J.ブイトラー、E.フェルフ、M.ファン・ドンケラー、R.プロミン、K.リムフェルト、S.E.フィッシャー、 B.St Pourcain
精神障害の多遺伝子リスクは、一般集団の社会的行動によって異なる関連プロファイルを明らかにする
Mol Psychiatr, 273 (2022), pp.1588-1598
2022
CrossRefScopusGoogle Scholarで表示する。
6
D.A.ピッツァガッリ
うつ病、ストレス、快感消失:統合と統合モデルに向けて
Annu Rev Clin Psychol, 10 (2014), pp. 393-423
クロスリーフScopusGoogleスカラーで見る
7
M.D.フィーリウ、C.サンディ
不安と脳内ミトコンドリア:双方向のクロストーク
Trends Neurosci, 42 (2019), pp.573-588
PDFを見る記事を見るScopusGoogle Scholarで見る
8
M. ピカール、C.サンディ
ミトコンドリアの社会性:人間の健康への影響
Neurosci Biobehav Rev (2021), p. 120
CrossRefScopusGoogle Scholarで表示する。
9
M. ピカール、B.S.マキューエン、E.S.エペル、C.サンディ
ストレスのエネルギッシュな見方:ミトコンドリアに着目して
フロント・ニューロエンドクリノール, 49 (2018)
グーグル スカラー
10
M.A.ファン・デル・クーイ、C.サンディ
社会的ヒエラルキーの遺伝学
Curr Opin Behav Sci, 2 (2015), pp.52-57
PDFを見る記事を見るScopusGoogle Scholarで見る
11
D.I.ベイダーベック、S.O.レバー、A.ハバシ、R.ブレデウォルド、A.H.ヴィーンマ、I.D.ニューマン
不安形質が極端に異なるラットの高攻撃性と異常攻撃性-側坐核のドーパミン系が関与している。
精神神経内分泌学, 37 (2012), pp.1969-1980
PDFを見る記事を見るScopusGoogle Scholarで見る
12
F. ホリス、M.A.ヴァン・デル・クーイ、O.ザノレッティ、L.ロザーノ、C.カントー、C.サンディ
脳内ミトコンドリア機能は不安と社会的従属を結びつける
Proc Natl Acad Sci U S A, 112 (2015), pp.15486-15491
CrossRefScopusGoogle Scholarで表示する。
13
E. ゲバラ、O・ザノレッティ、S・ゴーサル、J・グロッセ、B・L・シュナイダー、G・ノット、S・アストリ、C・サンディ
側坐核のミトフシン-2はミトコンドリアと神経細胞の作用により不安とうつ様行動を制御する
Biol Psychiatr, 89 (2021), pp.1033-1044
PDFを見る記事を見るScopusGoogle Scholarで見る
14
C.A.カラルコ、M.K.ロボ
個性的なパワーハウス:ミトフシン-2が特性不安の個人差に及ぼす側坐核ミトコンドリアへの影響を制御する
Biol Psychiatr, 89 (2021), pp.1024-1026
PDFを見る記事を見るScopusGoogle Scholarで見る
15
M.A. Van Der Kooij、F. Hollis、L. Lozano、I. Zalachoras、S. Abad、O. Zanoletti、J. Grosse、 I. Guillot De Suduiraut、 C. Canto、 C. Sandi
VTAにおけるジアゼパムの作用は、ドーパミンD1受容体の活性化により社会的優位性と側坐核のミトコンドリア機能を高める
モル・サイキアトル, 23 (2018), pp.569-578
CrossRefScopusGoogle Scholarで表示する。
16
L.A. Gunaydin, L. Grosenick, J.C. Finkelstein, I.V. Kauvar, L.E. Fenno, A. Adhikari, S. Lammel, J.J. Mirzabekov, R.D. Airan, K.A. Zalocusky, et al.
社会的行動の基盤となる自然な神経投射ダイナミクス
セル, 157 (2014)
グーグル スカラー
17
W. リー、T.M.マイルスキー、M.F.ドウォルツ、R.L.ヤング、A.D.ゴーデ、L.K.フォンケン、F.A.シャンパーニュ、J.P. カーリー
マウスの社会階層における支配的なオスと従属的なオスで異なる免疫およびトランスクリプトームプロファイルが見られる
Brain Behav Immun (2022), p. 103
PDFを見る記事を見るCrossRefを見るScopusGoogle Scholarで見る
18
S.E.ボーダー、T.J.ピーフケ、T.R.ファンネル、R.F.フィアルコウスキー、J.サベッキ、P.D.ダイクストラ
社会的不安定性がシクリッド魚の酸化ストレスのランク別パターンに影響を及ぼす
J Exp Biol (2021), p. 224
グーグル スカラー
19
D.J.コスティニーク、D.チャン、C.J.マーティニーク、K.M.ギルモア、J.A.メンニゲン
社会的地位はニジマスの肝臓miRNAomeを制御する:代謝経路の転写後制御への示唆
PLoS One, 14 (2019)
グーグル スカラー
20
R. デブレイ、N. スナイダー・マックラー、J.N. コーン、M.E. ウィルソン、L.B. バレイロ、J. トゥン
社会的所属はアカゲザルの雌におけるミトコンドリアDNAコピー数を予測する
バイオル・レット, 15 (2019)
グーグル スカラー
21
T. ヤルデニ、A.G.クリスタンチョ、A.J.マッコイ、P.M.シェーファー、M.J.マクマナス、E.D.マーシュ、D.C.ウォレス
ミトコンドリア欠損が自閉症のエンドフェノタイプをもたらすことをmtDNA変異マウスが証明した
Proc Natl Acad Sci U S A (2021), p. 118
グーグル・スカラー
22
F. デュクロ、L.セーラ、P.コウタキス、Z.ワン、M.カッバジ
社会的一夫一婦制をとるプレーリーボウルにおけるペアボンド形成と維持の基盤となるトランスクリプトーム規制
Biol Psychiatr (2022), p. 91
Google Scholar
23∗∗
A.K. Kanellopoulos, V. Mariano, M. Spinazzi, Y.J. Woo, C. McLean, U. Pech, K.W. Li, J.D. Armstrong, A. Giangrande, P. Callaerts, et al.
アララール、GABAを高活性ミトコンドリアに封じ込め、社会的行動障害を引き起こす
セル, 180 (2020), pp.1178-1197
ScopusGoogleスカラーで見る
自閉症や統合失調症に関連する遺伝子であるCYFIP1を変異させたショウジョウバエの社会的障害が、ミトコンドリアへのGABAの取り込みによって引き起こされ、このプロセスがトランスポーターAralarによって駆動されていることを実証した。
24
F. パルミエリ
ミトコンドリアトランスポーターファミリーSLC25:同定、特性、生理学的病理学
モル・アスペクト・メッド, 34 (2013), pp.465-484
PDFを見る記事を見るScopusGoogle Scholarで見る
D. D. Jimenez-Blasco、A. Busquets-Garcia、E. Hebert-Chatelain、R. Serrat、C. Vicente-Gutierrez、 C. Ioannidou、 P. Gómez-Sotres、 I. Lopez-Fabuel、 M. Resch-Beusher、 E. Resel, et al.
グルコース代謝がアストログリアミトコンドリアをカンナビノイド効果に関連付ける
ネイチャー, 583 (2020)
グーグル・スカラー
mtCB1受容体を介したミトコンドリア複合体I機能の低下により誘導されたアストロサイトの低ROSが、乳酸産生の低下をもたらすことを示す。その結果、神経細胞で酸化還元ストレスが生じ、その結果、社会的行動に支障をきたすことがわかった。
26
A. マイヤー-リンデンベルグ、C.B.メルヴィス、K.フェイス・バーマン
ウィリアムズ症候群の神経機構:認知と行動に対する遺伝的影響へのユニークな窓
Nat Rev Neurosci, 7 (2006), pp.380-393
CrossRefScopusGoogle Scholarで表示する。
27
S.J. Sanders, A.G. Ercan-Sencicek, V. Hus, R. Luo, M.T. Murtha, D. Moreno-De-Luca, S.H. Chu, M.P. Moreau, A.R. Gupta, S.A. Thomson, and al.
7q11.23ウィリアムズ症候群領域の重複を含む複数の再発性de novo CNVは自閉症と強く関連している
Neuron, 70 (2011), pp.863-885
PDFを見る記事を見るScopusGoogle Scholarで見る
28
A.T.N. Tebbenkamp, L. Varela, J. Choi, M.I. Paredes, A.M. Giani, J.E. Song, M. Sestan-Pesa, D. Franjic, A.M.M. Sousa, Z.W. Liu, and al.
7q11.23タンパク質DNAJC30はATP合成酵素と相互作用し、ミトコンドリアと脳の発達を結びつける
セル(2018)、175頁
グーグル スカラー
29
P.S. Ariyannur、J.R. Moffett、P. Manickam、N. Pattabiraman、P. Arun、A. Nitta、T. Nabeshima、C. N. Madhavarao、 A.M.A. Namboodiri
メタンフェタミン誘導性神経細胞タンパク質NAT8LはNAA生合成酵素である:中枢神経系における特殊なアセチルコエンザイムA代謝への示唆
ブレイン・レズ, 1335 (2010), pp.1-13
PDFを見る記事を見るScopusGoogle Scholarで見る
30
T.L. White, M.A. Gonsalves, R.A. Cohen, A.D. Harris, M.A. Monnig, E.G. Walsh, A.Z. Nitenson, E.C. Porges, D.G. Lamb, A.J. Woods, and al.
ウェルネスの神経生物学:健康な若年成人におけるエージェンシー、柔軟性、神経感情予備軍の1H-MRS相関関係
ニューロイメージ, 225 (2021)
グーグル・スカラー
31
E. リカン、P.デュベルジェ、C.カリウ、M.バース、C.プルートー、P.ヴァンボガート、D.ボノー、A.ロワ
ミトコンドリア病に罹患した小児・青年の神経心理学的・精神医学的特徴:システマティックレビュー
Front Psychiatr, 11 (2020), p. 747
ScopusGoogleスカラーで見る
32
S. ローズ、D.M.ニヤゾフ、D.A.ロシニョール、M.ゴールデンタール、S.G.カーラー、R.E.フライー
自閉症スペクトラム障害におけるミトコンドリア機能不全の臨床的・分子的特徴
Mol Diagn Ther, 225 (2018), pp.571-593
2018, 22
CrossRefScopusGoogle Scholarで表示する。
33
J. アレン、R.ロメイ=タロン、K.J.ブライマー、H.J.カランコ、L.E.カリンチュク
ミトコンドリアと気分:うつ病の顕在化におけるキープレイヤーとしてのミトコンドリア機能不全
フロント・ニューロサイ, 12 (2018)
グーグル・スカラー
34
A. デュプレ、J.オウム、I.エイベン、P.エイベン、P.A.ズンスザイン、C.M.パリアンテ、S.テュレ、C.フェルナンデス
齧歯類モデルにおいて、ストレスの種類によってうつ病に関連する行動および神経生物学的転帰が異なるか?システマティックレビュー
Front Neuroendocrinol, 61 (2021), 記事 100896
PDFを見る記事を見るScopusGoogle Scholarで見る
35
A. パピユー、I.ギヨ・ド・スデュイラート、O.ザノレッティ、J.グロッセ、C.サンディ
思春期前後のストレスは思春期における喧嘩ごっこを増加させ、側坐核CB1受容体の発現と扁桃体のミトコンドリア機能を変調させる
トランスル・サイキアトリー, 8 (2018)
グーグル スカラー
L. Morató, S. Astori, I. Zalachoras, J. Rodrigues, S. Ghosal, W. Huang, I.G. De Suduiraut, J. Grosse, O. Zanoletti, L. Cao, et al.
側坐核NAD+/SIRT1を介したENAMPTの作用により、脂肪率の上昇と思春期ストレスによってプログラムされる社交性障害とが関連付けられる
サイ・アド, 8 (2022)
Google Scholar
思春期前後のストレスによる脂肪率の上昇とアディポカインeNAMPTの減少が、生涯にわたる社交性障害に寄与することを実証。ENAMPTはNAcのNAD+/SIRT1経路に影響を与え、社交性をプログラムしている。
37
S. S. Alhassen, S. Chen, L. Alhassen, A. Phan, M. Khoudari, A. De Silva, H. Barhoosh, Z. Wang, C. Parrocha, E. Shapiro, et al.
世代間トラウマの伝達は、脳のメタボトランスクリプトームのリモデリングとミトコンドリア機能障害と関連している
Commun Biol (2021), p. 4
Google Scholar
38
H. Amini-Khoei, A. Mohammadi-Asl, S. Amiri, M.J. Hosseini, M. Momeny, M. Hassanipour, M. Rastegar, A. Haj-Mirzaian, A.H. Mirzaian, H. Sanjarimoghaddam, et al.
オキシトシンは、ミトコンドリア機能と神経炎症の調節を通じて、母体分離ストレスによる抑うつ様行動を緩和する。
Prog Neuro-Psychopharmacol Biol Psychiatry, 76 (2017), pp.169-178
PDFを見る記事を見るScopusGoogle Scholarで見る
39
S.R. Ruigrok, K. Yim, T.L. Emmerzaal, B. Geenen, N. Stöberl, J.L. den Blaauwen, M.R. Abbink, A.J. Kiliaan, E.M. van Schothorst, T. Kozicz, et al.
雄マウスの末梢および中枢ミトコンドリアに対する早世ストレスの年齢を超えた影響
精神神経内分泌学(2021年)、132頁
Google Scholar
K. Duan, Q. Gu, R.S. Petralia, Y.X. Wang, D. Panja, X. Liu, M.L. Lehmann, H. Zhu, J. Zhu, Z. Li
扁桃体基底部におけるミトファジーは、回避的な社会的経験によって誘発される不安の増大を媒介する
Neuron, 109 (2021), pp.3793-3809
Scopusで見るGoogle Scholar
社会的敗北ストレス感受性とBLAにおけるmtDNAコピー数変異の増加およびマイトファジーの関連性。BLAでマイトファジーを破壊すると、不安様表現型が救済される。
41
L.D. Zorova, V.A. Popkov, E.Y. Plotnikov, D.N. Silachev, I.B. Pevzner, S.S. Jankauskas, V.A. Babenko, S.D. Zorov, A.V. Balakireva, M. Juhaszova, and al.
ミトコンドリア膜電位
アナログバイオケム(2018)、552頁
グーグル スカラー
42
L. Guo, Z mei Jiang, R xue Sun, W. Pang, X. Zhou, M ling Du, Chen M. xiang, X. Lv, J tao Wang
社会的敗北を繰り返すストレスは、マイトファジーとオートファジーを介して海馬ニューロンの発達を阻害する
ブレイン・レズ・ブル (2022), p.182
Google Scholar
43
D.L.ウォーカー、D.J.トゥーフェクシス、M.デイヴィス
恐怖、ストレス、不安における線条体終末床核と扁桃体との役割について
Eur J Pharmacol, 463 (2003)
Google Scholar
44
Z. ミシェヴィッチ、S. イウラト、N. クレスカヤ、L. サルミネン、L. ロドリゲス、G. マッカローネ、J. マーティンス、D. チャマラ、M. A. レイン、E. ソコロフスカ、et al.
マルチオミクス解析により、不安関連行動と関連するミトコンドリア経路が特定された
PLoS Genet、15 (2019)
グーグル・スカラー
45
N. ソランキ、A.サルヴィ、G.パツキ、S.サリム
酸化ストレスを調節することで、ラットのストレス誘発性行動・認知障害が緩和される
Int J Neuropsychopharmacol, 20 (2017年)
グーグル スカラー
46
B.N. Dulka、A.K. Bourdon、C.T. Clinard、M.B.K. Muvvala、S.R. Campagna、M.A. Cooper
メタボロミクスにより、ストレスレジリエンスに関連する明確な神経化学プロファイルが明らかになった
ニューロバイオル・ストレス, 7 (2017)
グーグル スカラー
47
A.P. ミランダ・メンドンサ、L.Y. ホッペ、A. ガビラギ、TC デ アラウージョジョージ、G.M. デ オリベイラ、R.M. フェリッペ、M.F. オリベイラ、V.M. ダ シルバ フラゴーゾ
社会的ストレスにより誘発される高攻撃性行動は、マウス脳皮質におけるシトクロムcオキシダーゼ活性の低下と関連している
ニューロケム イント, 126 (2019)
グーグル・スカラー
48
M. ボベ、A.ラマ、S.スキアヴォーネ、C.ピロッツィ、P.トゥッチ、V.シコラ、G.トリンチェーゼ、G.コルソ、M.G.モージェーゼ、L.トラバチェ
社会的孤立は酸化状態を誘発し、正常血糖値ラットの全身および肝インスリン感受性を損なう
Biomed Pharmacother (2022), p.149
Google Scholar
49
M. シャルマ、S. カーン、S. ラーマン、L.R. シン
細胞外タンパク質であるトランスサイレチンは酸化ストレスバイオマーカーである
フロントフィジオール, 10 (2019)
グーグル スカラー
50
I. ペリッチ、V. コスティナ、P. ガス、P. フィンデイゼン、D. フィリポヴィッチ
雄ラットの慢性社会的孤立に対する感受性と回復力の海馬シナプトプロテオーム変化
ブレイン・レズ・ブル (2021), p.166
グーグル・スカラー
51
D. フィリポヴィッチ、I. ペリッチ、V. コスティナ、A. スタニサヴリェヴィッチ、P. ガス、P. フィンデイゼン
社会的孤立ストレスに強いラットは、海馬非シナプスミトコンドリアにおける解糖から酸化的リン酸化へのエネルギーシフトを明らかにした
ライフサイ (2020), p.254
グーグル・スカラー
52
M.P. Cunha, D.G. Machado, G. Mancini, V. Glaser, R. de Paula Martins, A.F. de Bem, A. Latini, A.L. Dafre, A.L.S. Rodrigues
自発的な輪行が抗酸化状態に及ぼす影響は、社交性条件に依存する
Pharmacol Biochem Behav (2020), p.198
グーグル・スカラー
53
L. ファン、ヤン、リー、テン、シアン、リュー、ジャン、朱、周、謝、ピー
慢性社会的敗北ストレスラットにおける扁桃体のプロテオームおよびメタボローム特性評価
Behav Brain Res (2021), p. 412
Google Scholar
54
L. 劉、X. 周、Y. 張、J. プー、L. ヤン、S. 元、L. ザオ、C. 周、H. 張、P. 謝
海馬の代謝の違いは、4つのうつ病モデルラットにおける身体的ストレスと心理的ストレスの区別に関与する
Transl Psychiatry, 8 (2018)
グーグル スカラー
55
Y. Zhu, Z. Fan, Q. Zhao, J. Li, G. Cai, R. Wang, Y. Liang, N. Lu, J. Kang, D. Luo, et al.
脳型グリコーゲンホスホリラーゼは、慢性社会的敗北ストレス誘発性うつ病マウスにおけるアストロサイトのグリコーゲン蓄積に重要である
Front Mol Neurosci (2022), p. 14
クロスリファレンス Google Scholar
56∗
P.J. Hamilton, E.Y. Chen, V. Tolstikov, C.J. Peña, J.A. Picone, P. Shah, K. Panagopoulos, A.N. Strat, D.M. Walker, Z.S. Lorsch, et al.
慢性的なストレスと抗うつ剤治療がマウスの脳と血清内のプリン代謝とβ酸化を変化させる
Sci Rep (2020), p. 10
グーグル・スカラー
慢性的な社会的敗北の影響は、異なる脳領域で均質ではないことを下敷きにしている。vHIP、mPFC、NAcで変化した代謝物の多くは、β酸化や抗酸化に関与したり、エネルギー代謝に関連するものだった。
57
A. Cherix, T. Larrieu, J. Grosse, J. Rodrigues, B. McEwen, C. Nasca, R. Gruetter, C. Sandi
アセチル-L-カルニチンの抗うつ剤様作用に関する側坐核の代謝シグネチャー
エライフ, 9 (2020)
グーグル スカラー
58
I. ペリッチ、V.コスティナ、S.ジョルジェヴィッチ、P.ガス、P.フィンデイゼン、D.インタ、S.ボルグワルト、D.フィリポヴィック
社会的に孤立したラットにおいて、ティアネプチンはシナプス小胞の動態を変調させ、シナプスミトコンドリアのプロセスを促進する
サイレポ, 11 (2021), pp.1-15
Scopusで見るGoogle Scholar
59
J.D. Kim、N.A. Yoon、S. Jin、S. Diano
ミクログリアのUCP2が高脂肪食による炎症と肥満を媒介する
セルメタボル, 30 (2019), pp.952-962
Scopusで見るGoogle Scholar
60
F.R.ジャルディム、F.T.デ・ロッシ、M.X.ナシメント、R.G.ダ・シルバ・バロス、P.A.ボージェ、I.C.プレシリオ、M.R.デ・オリベイラ
レスベラトロールと脳ミトコンドリア:レビュー
モル・ニューロバイオール、55 (2018)
グーグル スカラー
61
F. ホリス、E.S.ミッチェル、C.カント、D.ワング、C.サンディ
中鎖トリグリセリド食はラットの不安様行動を軽減し、社会的競争力を高める
ニューロファーマコロジー, 138 (2018)
グーグル スカラー
62
W. ワン、T. ワン、S. バイ、Z. チェン、X. チー、P. シュエ
Dl-3-n-ブチルフタライドはAKT/CREBシグナル経路を介したエネルギー代謝の制御により、慢性社会的敗北ストレスに対するマウスの行動障害を減衰させる
Transl Psychiatry, 10 (2020)
Google Scholar
63
A.P. Toniazzo, D.M. Arcego, C. Lazzaretti, C. Mota, C.E. Schnorr, L.F. Pettenuzzo, R. Krolow, J.C. Fonseca Moreira, C. Dalmaz
思春期前のストレスと高脂肪食へのアクセスによって誘発される視床下部のミトコンドリアおよび酸化ストレスパラメータに対する性差の影響
ニューロケム イント, 124 (2019)
グーグル・スカラー
64
R.C.フロムケ、L.J.ヤング
オキシトシン、神経可塑性、社会的行動
Annu Rev Neurosci (2021), p. 44
グーグル・スカラー
65
J. 趙、D. Qu、Z. Xi、Y. Huan、K. Zhang、C. Yu、D. Yang、J. Kang、W. Lin、S. Wu, et al.
ミトコンドリア移植は、神経細胞の生存とアストロサイトBDNFの促進を介して外傷性脳損傷を保護する
Transl Res (2021), p. 235
クロスレフグーグルスカラー
66
G・ジャヴァニ、S・バブリ、F・ファラジドクト、A・ガファリ=ナサブ、G・モハデス
ミトコンドリア移植はストレス曝露した高齢ラットの不安およびうつ病様行動を改善する
Mech Ageing Dev (2022), p. 202
Google Scholar
67
H. アイナット、P.ユアン、H.K.マンジ
Bcl-2遺伝子標的変異マウスにおける不安様行動の増加とミトコンドリア機能障害:不安障害におけるミトコンドリア機能の関与のさらなる支持
Behav Brain Res, 165 (2005)
Google Scholar
68
N.S.チャンデル
シグナル伝達オルガネラとしてのミトコンドリアの進化
セル・メタボル, 22 (2015), pp.204-206
PDFを見る記事を見るScopusGoogle Scholarで見る
69
M. ジャコメッロ、A.ピャクレル、C.グリツォ、L.スコラーノ
ミトコンドリア膜ダイナミクスの細胞生物学的研究
Nat Rev Mol Cell Biol, 214 (2020), pp.204-224
2020, 21
CrossRefScopusGoogle Scholarで表示する。
70
A. ミッドザック、V. パパドプロス
副腎ミトコンドリアとステロイド生成:個々のタンパク質から機能的なタンパク質集合体へ
フロントエンドクリノール, 7 (2016)
グーグル スカラー
71
H. チェン、S.A.デトマー、A.J.エワルド、E.E.グリフィン、S.E.フレーザー、D.C.チェン
ミトフシンMfn1とMfn2は、ミトコンドリア融合を協調的に制御し、胚発生に必須である。
J Cell Biol (2003), p. 160
Google Scholar
72
D.A. ターナー, D.C. アダムソン
神経細胞-アストロサイトの代謝相互作用:アストロサイトーマの代謝異常への移行を理解する
J Neuropathol Exp Neurol, 70 (2011)
Google Scholar
73
D. ノルフィ・ドネガン、A. ブラガンザ、S. シバ
ミトコンドリア電子輸送鎖:酸化的リン酸化、酸化剤生成、測定方法について
レドックス・バイオル(2020)、37頁
グーグル・スカラー
74
M. Sharifi-Rad, N.V. Anil Kumar, P. Zucca, E.M. Varoni, L. Dini, E. Panzarini, J. Rajkovic, P.V. Tsouh Fokou, E. Azzini, I. Peluso, et al.
ライフスタイル、酸化ストレス、抗酸化物質:慢性疾患の病態生理における行きつ戻りつ
フロントフィジオール(2020)、11頁
グーグル・スカラー
引用元: (1)
長時間のパートナーとの別離は、オスのプレーリーハタネズミのペアボンディングの側坐核転写シグネチャーを侵食する
2023年、イーライフ
a
著者は平等に貢献した。
© 2023 The Authors. Elsevier Ltd.が発行しています。
ScienceDirectについて
リモートアクセス
ショッピングカート
広告掲載
お問い合わせ・サポート
ご利用条件
個人情報保護方針
当社は、サービスの提供や強化、コンテンツや広告のカスタマイズのためにCookieを使用しています。継続することで、クッキーの使用に同意することになります。
Copyright © 2023 Elsevier B.V.またはそのライセンサーもしくは寄稿者。ScienceDirect® はElsevier B.V.の登録商標です。
Powering the social brain: Mitochondria in social behaviour
Author links open overlay panelDoğukan Hazar Ülgen a, Silvie Rosalie Ruigrok a, Carmen Sandi
Show moreOutlineShareCite
https://doi.org/10.1016/j.conb.2022.102675Get rights and content
Under a Creative Commons license
open access
Highlights
•
Mitochondrial variation in specific brain regions is associated with individual differences in sociability and social status.
•
Stress-induced sociability deficits entail mitochondrial dysfunctions.
•
Mitochondrial dysfunctions encompass changes in energy metabolism, oxidative damage, and/or compromised lipid metabolism.
•
Nutritional supplementation targeting mitochondria emerges as a promising intervention strategy to tackle social dysfunction.
Abstract
A central role of brain mitochondria in regulating and influencing social behaviour is emerging. In addition to its important roles as the “powerhouses” of the cell, mitochondria possess a plethora of cellular functions, such as regulating ion homeostasis, neurotransmitter levels, and lipid metabolism. Findings in the last decade are revealing an integral role for mitochondria in the regulation of behaviours, including those from the social domain. Here, we discuss recent evidence linking mitochondrial functions and dynamics to social behaviour and deficits, including examples in which social behaviours are modulated by stress in the context of mitochondrial changes, as well as potential therapeutic strategies and outstanding questions in the field.
Introduction
Across the animal kingdom, many species, including humans, have evolved to be social, as - among other reasons - being alone in an environment full of predators poses numerous dangers. Therefore, our brains - and those of many other species - are extensively social. This is reflected in the plethora of brain regions and interconnected circuits involved in the social domain, including different hypothalamic nuclei, the nucleus accumbens (NAc), ventral tegmental area (VTA), amygdala, hippocampus (HIP) and prefrontal cortex (PFC); ranging from those engaged in the processing and consolidation of social cues, to those involved in reward, motivation, decision-making and action regulation [1,2]. Alterations in social behaviour are present in most mental health disorders, including depression, anxiety disorders and autism [3, 4, 5]. Frequently, these conditions involve reduced sociability, as well as trouble integrating in social groups, forming pair-bonds or aggression towards others. Chronic stress is an important risk factor for developing both aberrant social behaviours and mental health disorders [1,6]. Accordingly, understanding the neural mechanisms that govern social function and dysfunction is a critical step to discern the underpinnings of some of the most common mental health disorders, and may reveal novel avenues for therapeutic interventions.
Recent emerging evidence puts brain mitochondria under the spotlight for brain (dys)function [7,8], including psychopathologies that course with social alterations [9]. Mitochondria are double-membraned organelles crucial for cellular energy metabolism, as well as ion homeostasis, lipid metabolism and signal transductions (see Box 1). In this review, we focus on recent findings linking mitochondrial function and dynamics to social behaviour, together with examples in which social behaviours are modulated by stress in the context of mitochondrial changes, and discuss potential therapeutical strategies and future perspectives (Figure 1).
Box 1
Mitochondria functions and dynamics
Mitochondria are both bioenergetic and ‘signaling organelles’ [68]. The inner mitochondrial membrane is primarily involved in the production of adenosine triphosphate (ATP) through oxidative phosphorylation (OXPHOS). The outer membrane forms membrane contact sites with other organelles such as lysosomes and endoplasmic reticulum, and is the main platform for mitochondrial signaling, as well as critical for fission (division) and fusion of mitochondria [69]. Mitochondria undergo fusion to become large and do so when, for example, energy demand is high. Fission often occurs when mitochondria are damaged and should be targeted for mitophagy (the degradation of a mitochondrion). In addition, mitochondria synthesize steroid hormones, regulate apoptosis, and modulate the cellular ion homeostasis (reviewed in Refs. [69,70]). Their morphology is strongly related to their function [71]. Both their fusion/fission dynamics, overall morphology (length, width, roundness) and ultrastructural organization (inner and outer membranes) vary between different cell types, cell cycle stages and in response to metabolic signals.
Most cell types largely rely on OXPHOS in mitochondria for the production of ATP (e.g. neurons), though there are exceptions (e.g. astrocytes relying on glycolysis) [72]. Before OXPHOS can take place, glucose, fatty acids and/or amino acids need to be catabolized. The derived products enter the tricarboxylic acid (TCA) cycle in the mitochondria, which then produces substrates that are used for OXPHOS by the electron transport chain (ETC) located in the inner mitochondrial membrane [73]. The ETC comprises of four protein complexes (complex I-IV) through which electrons travel, creating a proton gradient (also known as the mitochondrial membrane potential). The OXPHOS process starts with the release of electrons produced by reducing TCA-derived NADH into NAD+ and ends with the addition of phosphate to adenosine diphosphate (ADP) by ATP synthase (complex V), to generate ATP. During OXPHOS, oxygen acts as an electron acceptor, and mitochondrial oxygen consumption can thus be used as a proxy for mitochondrial activity.
Reactive oxygen species (ROS) are produced as a natural byproduct during OXPHOS due to electron leakage, causing a reduction of oxygen to O2•-, which is usually rapidly converted into H2O2 by superoxide dismutase (SOD) (reviewed in depth in Ref. [74]). H2O2 can leave the mitochondrion and function as a signaling molecule, regulating processes such as cell growth and differentiation. Despite its physiological role, high levels of ROS can cause damage to the cell by oxidation of membrane lipids and DNA. Therefore, antioxidant systems, such as the glutathione (GSH) system, are set in place to protect from high ROS levels, and subsequently, cellular and mitochondrial damage.
Figure 1. Mitochondrial function and dynamics are central in social behaviour and stress-induced social deficits. Nutritional interventions targeting mitochondria modulate sociability and social status. ATP, adenosine triphosphate; ROS, reactive oxygen species; OXPHOS, oxidative phosphorylation; TCA, tricarboxylic acid; mtDNA, mitochondrial DNA.
Mitochondria and social competition
The formation of social hierarchies is crucial for maintaining group homeostasis, such as the division of labour and resources, and the odds of becoming dominant or subordinate in a social competition is - to some extent - biologically determined [10]. The first evidence indicating that mitochondrial function in specific brain regions could be critical for social behaviours was related to social competition. The nucleus accumbens (NAc) - a brain hub for motivated behaviour - had been shown to be critically involved in the outcome of a competitive encounter, as it is highly activated during exposure to contests and its inhibition reduces social dominance [11,12]. In 2015, Hollis et al. [12] identified a key role for mitochondria in the NAc in social competition. They showed that when two male rats of equal characteristics and life history, but differing in their anxiety temperament, competed for a new territory, high anxious (HA) rats were more prone to becoming subordinate than their low anxious (LA) opponents. Strikingly, these behavioural results were linked to a range of alterations in NAc neuronal and mitochondrial structure and function. As compared to LA, HA rats showed dendritic atrophy and reduced density of mature spines in medium spiny neurons along with a reduced frequency of excitatory inputs [13], denoting a reduced capacity of these neurons to get activated by glutamatergic afferents. These morphological differences were accompanied by an increased expression of mitochondria-related genes and, congruent with these findings, lower mitochondrial complex I and II protein levels (see Box 1), lower respiratory capacity and ATP levels, while higher ROS production, in the NAc of HA animals as compared to LA [12,13]. Importantly, boosting mitochondrial function by nicotinamide infusion in the NAc abolished the propensity to become subordinate in HA rats [12]. Although mitochondrial density in NAc neurons was similar in both anxiety groups, subsequent studies showed that mitochondrial morphology was drastically different: mitochondria were more globular and voluminous in HA rats and showed a much lower number of contacts with the endoplasmic reticulum (ER) than in LA animals [13]. Thus, proteins involved in mitochondrial dynamics and their interaction with the ER were potentially involved in the observed phenotypes. Further research underscored a causal role for reduced levels of mitofusin 2 (MFN2; a protein regulating mitochondrial fusion and mitochondria-ER interactions) in the NAc, in the mitochondrial, neuronal and behavioural phenotypes reported above for HA rats [13]. Altogether, these findings highlight a prominent role for natural variation in Mfn2 expression in the NAc in explaining individual differences in brain structure and function, as well as motivated behaviour [14].
This established rat model of anxiety and social competition also revealed that both social competition and NAc mitochondrial function can be modulated by treatment with the anxiolytic diazepam. Diazepam given to HA rats, either peripherally (at a low dose) or in the ventral tegmental area (VTA) - the brain region containing the dopaminergic neurons projecting to the NAc - reverted their competitive disadvantage against LA rats [15]. Importantly, this effect was shown to involve increased dopamine release into the NAc which, through the activation of dopamine receptor 1 (DRD1), could transiently boost mitochondrial function (i.e., respiration and ATP production). Blocking complex I-related mitochondrial respiration in the NAc prevented the effect of diazepam, eventually linking mitochondrial function in the NAc to dopamine signalling [15]. These findings are in line with earlier literature showing VTA-NAc projections to regulate social behaviour via NAc DRD1 signalling [16], and further expand on these by implicating a key role for mitochondria in this connection.
Next to identifying brain mitochondrial function to regulate social competitiveness in male rats, social status has also been related to mitochondrial function across several species in peripheral tissues. For example, subordinate mice showed higher expression of genes involved in oxidative phosphorylation in spleen, while dominants displayed increased expression of genes involved in carboxylic and lipid catabolic processes in liver [17]. In cichlid fish, dominant males showed increased plasma reactive oxygen species (ROS) levels compared to subordinates in stable – but not unstable – hierarchies [18]. In addition, liver gene expression analysis indicated more mitochondrial fission and less fusion in subordinate rainbow trout [19], while in female rhesus macaques, time spent grooming (a measure of affiliative behaviour indicative of higher rank), but not rank measured by agonistic behaviour, correlated positively to mtDNA copy number in B cells [20]. Together, these studies show social status-dependent variation in mitochondrial functions across different species and different tissues/cell types.
Mitochondria and sociability
Subsequent studies have shown that, beyond its role in social rank establishment, mitochondria are also involved in other aspects of social behaviour. For instance, mitochondrial deficits induced by a mutation in the mtDNA gene Nd6 have been associated with reduced sociability in a social preference task in male mice [21]. Interestingly, in social monogamous prairie voles, pair-bonding was accompanied by higher mtDNA copy numbers in the NAc of male - but not female - prairie voles, as well as sex-specific alterations in the expression of genes regulating mitochondrial fission (Fis1, Dnm1l), indicating increased fission in the NAc of males after cohabitation [22].
Recent work in invertebrates has provided further insights into the underlying mechanisms by which mitochondria may regulate social behaviour. Specifically, Drosophila carrying mutations in the homolog of the human CYFIP1, a protein regulating cytoskeletal dynamics and protein translation linked to autism and schizophrenia, showed deficits in social group behaviour (e.g. increased distance between flies within a social group and reduced courtship behaviour), which were accompanied by increased mitochondrial respiration, mitochondrion size, and higher TCA cycle activity in the brain [23]. Social deficits in mutant flies were causally linked to defects in GABA signalling, as GABA accumulated in mitochondria of mutants [23]. GABA uptake was driven by the mitochondrial transporter Aralar, a solute carrier located in the outer mitochondrial membrane, previously described to exchange aspartate for glutamate and a proton [24]. Alongside the involvement of GABAergic signalling in modulating social behaviour, energy metabolism in astrocytes has also been shown to affect social behaviour in male mice [25]. In fact, the cannabinoid-induced social deficit in a social preference task could be retraced to astrocytic mitochondria. Activating astroglial type-1 cannabinoid receptors associated with mitochondrial membranes (mtCB1) decreased the stability and activity of mitochondrial complex I, lowering ROS production in astrocytes. The low astrocytic ROS hampered lactate production in the astrocytes, causing redox stress in the neurons. Consequently, excessive ROS produced in neurons mediated the social interaction deficits induced by cannabinoids. Thus, mitochondria can regulate social behaviour directly by sequestering GABA in neurons, but also indirectly by lowering metabolic support from astrocytes to neurons.
In contrast to the reduced sociability occurring in mental disorders as anxiety, depression and autism, hypersociability is a prominent feature of Williams syndrome (WS) [26]. WS is caused by the deletion of 26–28 genes from chromosome 7 (7q11.23), while duplication of this region is associated with autism [27]. Notably, mitochondrial function has also been linked to WS pathogenesis and its associated increased sociability [28]. Fibroblasts from WS patients showed decreased mitochondrial respiration and ATP synthesis parallel to increased ROS production, in line with alterations in OXPHOS complex protein levels in the neocortex of WS patients. The 7q11.23 protein DNAJC30, an auxiliary component of the ATP synthase machinery, was identified to in part facilitate these mitochondrial alterations, as DNAJC30 knock out mice showed increased social interest alongside neuronal morphological and mitochondrial alterations.
As exemplified, both increased and decreased mitochondrial function are related to social deficits [23,25], while decreased mitochondrial function is also linked to hypersociability [28]. Therefore, the relationship between mitochondrial function and social behaviour seems not to be linear, but rather parabolic where either too little or too much activity can lead to abnormalities.
In humans, studying the relationship between social behaviour and brain mitochondrial function is challenging, since brain mitochondria are only accessible post-mortem. Brain metabolites measured with 1H-magnetic resonance spectroscopy can instead serve as a proxy for mitochondrial function and other metabolic processes in living individuals. Thus, recent work in healthy human subjects has shown an association between N-acetylaspartate (NAA; a storage form of acetyl coenzyme A - the main substrate entering the TCA cycle - that is produced in neuronal mitochondria [29]) levels in the dorsal anterior cingulate cortex and several emotional constructs, including social potency and aggression [30]. These data suggest that the link between brain energy metabolism and social behaviour described above in rodents also exists in humans. However, a note of caution should be added, as this study involved a rather small sample size. Importantly, in line with findings in animals in which mitochondrial functions are impaired through genetic mutations or pharmacological manipulations [12,13,15,21,23], human patients with mitochondrial diseases also often display psychiatric symptoms (reviewed in Ref. [31]). And, conversely, alterations in (brain) energy metabolism have been identified in psychopathological conditions involving changes in social behaviour, such as autism spectrum disorder (reviewed in Ref. [32]), as well as in depression (reviewed in Ref. [33]). However, whether variation in brain energy metabolism is also related to social behaviour under nonpathological conditions in humans requires further investigation.
Stress-induced sociability deficits can be traced back to mitochondria
Stress occurring at different times throughout individual's life can have a strong impact on social behaviour, not just in humans but in rodents as well [1]. Along with changes in mood, motivation or anxiety, both physical and psychosocial stress can lead to reduced sociability and increased aggression [1,34]. Mitochondrial changes in the brain are now being implicated in the effect of stress and the resulting sociability deficits. Here, we review evidence on both early-life and adulthood stress.
Early-life stress-induced programming of social behaviours – the mitochondria connection
Early-life stressful experiences can have a strong impact on social behaviour, frequently leading to decreased social motivation, development of antisocial features, and increased aggressiveness in adult life (reviewed in Ref. [1]). They can also affect the structure and function of several brain regions critically involved in the regulation of social behaviours, such as the PFC, HIP, and the hypothalamus; and these findings have been reported for both humans and rodents.
Furthermore, exposure to early-life stressful experiences can also program brain mitochondrial functions [35, 36∗∗, 37, 38, 39] and contemporary findings link those mitochondrial changes to stress-induced alterations in social behaviours. First, correlational evidence has been reported in studies showing that peripubertal stress in male rodents leads to increased aggression [35] and social deficits later in life [36], alongside mitochondrial dysfunctions in the amygdala (increased respiration) [35] and NAc (reduced respiration) [36], respectively. Second, both peripheral and brain energy metabolism have been causally linked to peripubertal stress-induced social deficits in a recent study [36]. Specifically, peripubertally stressed male, but not female, mice were found to concomitantly show deficits in sociability and increased adiposity at adulthood. Strikingly, a reduction in the levels of the adipokine nicotinamide phosphoribosyltransferase (NAMPT) in fat and its extracellular form eNAMPT in blood contributed to the reduced sociability. The observed decrease in eNAMPT contributed to a deficit in the levels of nicotinamide adenine dinucleotide (NAD+) in the NAc and, consequently, on the activity of the SIRT1 pathway. These alterations in turn led to a reduction in the firing frequency of the NAc medium spiny neurons and associated changes in sociability. Importantly, treatment with nicotinamide mononucleotide (NMN), a NAD+ booster, following exposure to peripubertal stress prevented the expression of social deficits at adulthood [36]. Besides peripubertal stress, pre- and early postnatal stress cause social deficits and depressive-like behaviour later in life as well, together with increased levels of the mitochondrial hypoxia marker 2-hydroxyglutaric acid [37]. Moreover, an intervention with acetyl-l-carnitine (ALC), a mitochondrial boosting compound, protected mice from developing depressive-like behaviour [37]. Together, these studies indicate that early-life experiences can program later life social behaviour, at least in part, through changes in mitochondria.
Changes in social behaviour upon stress at adulthood – the mitochondria connection
Exposure to stressors during adulthood can also affect social behaviours, frequently leading to social avoidance and fear towards unknown conspecifics [1]. Most of the studies implicating mitochondria in adult stress-induced sociability deficits utilize the social defeat paradigm. In male mice, social defeat was shown to cause a decrease in the mitochondrial size and mass in the basolateral and central nucleus of the amygdala [40]. Accordingly, the membrane potential of the mitochondria, which is harnessed to yield ATP [41], was also found to be reduced in these brain regions. The stress-susceptible animals showed an increase of mtDNA mutations and a decrease of mtDNA copy number, which was associated with elevated levels of mitophagy. Downregulating mitophagy abolished anxiety-like behaviours. Furthermore, mitochondria of the hippocampal neurons of stress-susceptible male mice were also found to be negatively affected by enhanced mitophagy and autophagy, resulting in disrupted neuronal growth and development [42]. Intact, functional mitochondria are essential for the normative function of the neurons, and mitophagy and autophagy provide a strenuous surveillance over the “quality” of mitochondria (box 1). A neuronal mitochondrion whose function is compromised by sub-optimal structure or mutated mtDNA, especially when it cannot be targeted by mitophagy, could affect the functioning of neural circuits through different mechanisms (e.g., by inducing cellular energy deficits, alterations in calcium buffering, neurotransmitter production, or other) eventually contributing to the stress-like phenotype.
Other studies have shown that multiple circuits can be affected by social defeat stress. To illustrate, an integrated multi-omics analysis from the bed nucleus of the stria terminalis of male C57BL/6 mice, another region associated with stress response [43], revealed that oxidative phosphorylation was upregulated, whereas another mitochondria-related pathway, SIRT1 signalling, was downregulated by social defeat stress [44]. Interestingly, the same pathways were found to be affected by social defeat in stressed DBA/2 male mice, as well, but in the opposite direction, emphasizing the inter-strain differences of gene regulation. Together, these findings highlight mitochondrial homeostasis as crucial for the social brain; disruptions in this fine balance can lead to, or be influenced by, stress-induced sociability defects.
Mitochondrial homeostasis is critically involved in the regulation of the cellular concentration of ROS. ROS arise as a by-product of oxidative phosphorylation, and while a certain level of them is beneficial to the cellular function, high levels can cause damage to DNA, protein, or lipids (see Box 1). Social defeat has been shown to lead to reduced protein levels of oxidative stress enzymes such as glutathione reductase 1, glyoxalase 1 and superoxide dismutase (SOD) in the HIP [45]. Moreover, stress-resistant animals were found to exhibit an increased concentration of cystine (a dimer of cysteine that can be converted to the antioxidant GSH) in the NAc and ventromedial PFC [46]. While the oxygen consumption rate and ATP synthesis did not show a difference in the PFC of aggressive mice, cytochrome c oxidase levels and activity were found to be lower [47]. This dysregulation was not confined to the brain, as ROS and SOD activity were also increased in the liver, along with decreased GSH, in socially-isolated rats [48]. In addition to changes in neuronal excitation produced by stress leading to increased ROS production, stress can affect oxidation through transcriptional effects of stress hormones. Notably, the transthyretin gene, whose mRNA and protein levels change in proportion to cellular oxidative stress, contains a glucocorticoid receptor response element in its first intron, and is found to be upregulated upon stress (reviewed in Ref. [49]). Together, these converging evidences suggest the involvement of increased ROS levels caused by mitochondrial dysfunction as an underlying mechanism of stress.
Mitochondrial dysfunction can have wide-spanning causes and effects; usually involving changes in the energy and lipid metabolism. Indeed, many proteomic, lipidomic and metabolic studies allude to potential disruptions upon stress. In male rats, social isolation leads to an increase in glucose metabolism and vesicle trafficking proteins in the synaptosomal fractions obtained from the HIP [50]. On the other hand, in the cytosolic fractions, a shift from glycolytic enzymes to oxidative phosphorylation has been observed, as well as a downregulation in mitochondria-related redox system and calcium signalling [51]. In male mice, social isolation induces lipid peroxidation in the PFC, suggesting an increase in energy demands [52]. In the amygdala and the PFC of male rats subjected to social defeat stress, NAA was found to be increased [53]. On the other hand, in the HIP of male rats exposed to social defeat, both lactic acid and NAA were increased, hinting to increased glycolysis and mitochondrial dysfunction, respectively [54]. Glycogen accumulation was observed in the medial PFC of the social defeat stress-susceptible mice, and astrocytic brain type glycogen phosphorylase PYGB dysfunction was sufficient to induce glycogen accumulation and depression-like behaviours observed upon social defeat stress [55]. Chronic social defeat stress led to strong and divergent changes in metabolites and lipids, in the ventral HIP, NAc and the mPFC [56]. Changing metabolites ranged from purine nucleotides, carnitine donors to modified fatty acids involved in beta oxidation, and antioxidants. Finally, LAC-supplemented mice survived the adverse effects of chronic restraint stress on high ranking mice [57]. Since LAC is involved in the transport of long-chain fatty acids into the mitochondria, facilitating this transport is likely to tip the energy metabolism in favour of lipid oxidation, thereby counterbalancing the glycolysis-promoting energy metabolism of stress [58]. Collectively, these findings emphasize that defects in energy and lipid metabolism, which may pave the way to, or have originated from, mitochondrial dysfunction, can spearhead the effects of social stress in the brain.
Nutritional intervention strategies
The fact that mitochondrial energy metabolism regulates social behaviour (e.g. Refs. [12,36]) and that diet and specific nutrients impact on brain mitochondrial functions [59,60], raises the question as to whether social behaviours can be modulated via dietary supplementation. A few emerging studies already support this view. For example, peripubertal stress-induced social deficits could be rescued by dietary supplementation of nicotinamide mononucleotide, a NAD+ booster [36]. Another study showed that supplementation of medium chain triglycerides (an effective energy source for mitochondria) increased social status in high anxious rats [61]. Improved mitochondrial function within the mPFC, but not the NAc, was associated with these dietary effects. Furthermore, supplementation with LAC, a mitochondrial booster, also exerted beneficial effects in high rank - but not low rank - mice exposed to chronic stress [57]. In fact, chronic stress exposure to high rank mice increased depressive-like behaviours and lowered the levels of metabolites such as phosphocreatine (involved in energy metabolism) and taurine (antioxidant), while LAC supplementation reversed the levels of these metabolites to control levels. Finally, intragastric Dl-3-n-Butylphthalide (NBP) administration, a synthetic compound based on l-3-n-Butylphthalide that is isolated from seeds of celery (Apium graveolens), mitigated stress-induced social deficits, anxiety-like behaviour and despair, as well as altered metabolite levels of glycolysis and the TCA cycle [62]. Thus, nutrition-based interventions serve a potential therapeutic target for (stress-induced) social deficits.
Conclusion and future perspectives
Mitochondria are emerging as a critical organelle in the regulation of social behaviours and, accordingly, we also attest here that changes in mitochondrial function are involved in the modulation of social behaviours by stress. Problems with brain mitochondria can manifest in various ways, from an imbalance in energy metabolism (glycolysis vs. oxidative phosphorylation), disrupted ion homeostasis and increased cellular ROS levels, to compromised lipid metabolism (beta-oxidation or peroxidation). Potential intervention strategies include NAD+ boosters, NBP or medium chain fatty acid supplementation.
There are many outstanding questions. Most of the preclinical studies are performed in males only. Only a handful of studies include both sexes, and differences in mitochondrial function between males and females have already been reported. For example, the susceptibility to ROS upon social isolation stress is higher in female than male mice [63], and mtDNA copy number increased only in pair-bonded male, but not female, prairie voles [22]. Thus, whether similar brain circuits, cellular and molecular processes are involved in female social behaviour needs further investigation.
Due to the interconnected nature of social behaviour, both on the level of the numerous brain regions involved as well as the peripheral and hormonal regulation, it has thus far been a challenge to delineate the role of mitochondria in these different aspects of behavioural responses. For example, dopamine is known to modulate social behaviour, and increased dopamine signalling in the NAc has been shown to boost mitochondrial function, leading to changes in the behavioural output [15]. It would be imperative to extend this investigation into other behaviour-regulating hormones, such as oxytocin [64], and to understand which of these links between hormones and behaviour has mitochondrial foundations.
As outlined throughout this review, mitochondrial function and dynamics have been implicated in many different aspects of social behaviour, as well as anxiety- and stress-related changes in behaviour. However, the mitochondrial changes could either be i) a primary driving force of variation in social behaviour, or rather ii) a secondary effect to another underlying, (neuro)biological difference, such as dysregulated transcription due to a mutated transcription factor, fundamental differences in the chromatin topology or circulating factors. Evidence for mitochondria being a primary substrate to regulate behaviour comes from recent studies showing that intracerebroventricular mitochondrial transplantation improved anxiety- and depression-like behaviour in mice and rats, respectively [65,66]. Another study illustrated that a mutation in the Bcl-2 protein, which is situated in the inner mitochondrial membrane and modulates the Ca2+ uptake by the mitochondria, caused mice to exhibit more anxiety-like behaviours [67]. On the other hand, reduced levels of the adipokine NAMPT in the blood was shown to underlie the behavioural abnormalities and mitochondrial defects in the NAc in a peripubertal stress model, and repletion of NAMPT levels rescued these effects [36]. Considering how central mitochondria are to cellular -and thus brain-function, we think that both views presented here hold truth; while mitochondrial changes may be the driving force behind some behavioural deficits, they may also be secondary to other neurobiological differences in others. Associating specific mitochondrial abnormalities (i.e., mutations of a mitochondrial gene) with certain behavioural disorders, followed by modelling these abnormalities to replicate the disorder in transgenic animal models will be able to shed some light into this discussion.
Another critical question is to understand whether there are cell type-specific differences in the mitochondrial function that govern social behaviour. While a few studies report such cell type specificity [25,55], further research is imperative to unveil the specific contribution of mitochondria to social behaviour in different cell types. Finally, given the metabolic functions and dependence of mitochondria on substrates - as well as their products - amenable to nutritional or pharmacological targeting, emerging findings in this field bring promise to investigate the therapeutic potential of mitochondria-targeting approaches.
Conflict of interest statement
Nothing declared.
Acknowledgements
This work was supported by grants from the Swiss National Science Foundation (SNSF) [176206 and 197942; NCCR Synapsy: 51NF40-158776 and −185897], ERA-NET (Biostress; SNSF No 31NE30_189061) and intramural funding from the EPFL to C.S. SR is funded by the SNSF Swiss Postdoctoral Fellowships (grant number TMPFP3_210670).
Special issue articlesRecommended articles
Data availability
No data was used for the research described in the article.
References
1C. Sandi, J. HallerStress and the social brain: behavioural effects and neurobiological mechanismsNat Rev Neurosci, 16 (2015)Google Scholar
2S.D. Lanooij, U.L.M. Eisel, W.H.I.M. Drinkenburg, E.A. van der Zee, M.J.H. KasInfluencing cognitive performance via social interactions: a novel therapeutic approach for brain disorders based on neuroanatomical mapping?Mol Psychiatr (2022), 10.1038/s41380-022-01698-1Google Scholar
3I.M.J. Saris, M. Aghajani, S.J.A. van der Werff, N.J.A. van der Wee, B.W.J.H. PenninxSocial functioning in patients with depressive and anxiety disordersActa Psychiatr Scand, 136 (2017), pp. 352-361CrossRefView in ScopusGoogle Scholar
4U. Frith, F. HappéAutism spectrum disorderCurr Biol, 15 (2005), pp. R786-R790View PDFView articleView in ScopusGoogle Scholar
5F. Schlag, A.G. Allegrini, J. Buitelaar, E. Verhoef, M. van Donkelaar, R. Plomin, K. Rimfeld, S.E. Fisher, B. St PourcainPolygenic risk for mental disorder reveals distinct association profiles across social behaviour in the general populationMol Psychiatr, 273 (2022), pp. 1588-15982022CrossRefView in ScopusGoogle Scholar
6D.A. PizzagalliDepression, stress, and anhedonia: toward a synthesis and integrated modelAnnu Rev Clin Psychol, 10 (2014), pp. 393-423CrossRefView in ScopusGoogle Scholar
7M.D. Filiou, C. SandiAnxiety and brain mitochondria: a bidirectional crosstalkTrends Neurosci, 42 (2019), pp. 573-588View PDFView articleView in ScopusGoogle Scholar
8M. Picard, C. SandiThe social nature of mitochondria: implications for human healthNeurosci Biobehav Rev (2021), p. 120CrossRefView in ScopusGoogle Scholar
9M. Picard, B.S. McEwen, E.S. Epel, C. SandiAn energetic view of stress: focus on mitochondriaFront Neuroendocrinol, 49 (2018)Google Scholar
10M.A. van der Kooij, C. SandiThe genetics of social hierarchiesCurr Opin Behav Sci, 2 (2015), pp. 52-57View PDFView articleView in ScopusGoogle Scholar
11D.I. Beiderbeck, S.O. Reber, A. Havasi, R. Bredewold, A.H. Veenema, I.D. NeumannHigh and abnormal forms of aggression in rats with extremes in trait anxiety--involvement of the dopamine system in the nucleus accumbensPsychoneuroendocrinology, 37 (2012), pp. 1969-1980View PDFView articleView in ScopusGoogle Scholar
12F. Hollis, M.A. Van Der Kooij, O. Zanoletti, L. Lozano, C. Cantó, C. SandiMitochondrial function in the brain links anxiety with social subordinationProc Natl Acad Sci U S A, 112 (2015), pp. 15486-15491CrossRefView in ScopusGoogle Scholar
13E. Gebara, O. Zanoletti, S. Ghosal, J. Grosse, B.L. Schneider, G. Knott, S. Astori, C. SandiMitofusin-2 in the nucleus accumbens regulates anxiety and depression-like behaviors through mitochondrial and neuronal actionsBiol Psychiatr, 89 (2021), pp. 1033-1044View PDFView articleView in ScopusGoogle Scholar
14C.A. Calarco, M.K. LoboThe individualized powerhouse: mitofusin-2 regulates nucleus accumbens mitochondrial influence on individual differences in trait anxietyBiol Psychiatr, 89 (2021), pp. 1024-1026View PDFView articleView in ScopusGoogle Scholar
15M.A. Van Der Kooij, F. Hollis, L. Lozano, I. Zalachoras, S. Abad, O. Zanoletti, J. Grosse, I. Guillot De Suduiraut, C. Canto, C. SandiDiazepam actions in the VTA enhance social dominance and mitochondrial function in the nucleus accumbens by activation of dopamine D1 receptorsMol Psychiatr, 23 (2018), pp. 569-578CrossRefView in ScopusGoogle Scholar
16L.A. Gunaydin, L. Grosenick, J.C. Finkelstein, I.V. Kauvar, L.E. Fenno, A. Adhikari, S. Lammel, J.J. Mirzabekov, R.D. Airan, K.A. Zalocusky, et al.Natural neural projection dynamics underlying social behaviorCell, 157 (2014)Google Scholar
17W. Lee, T.M. Milewski, M.F. Dwortz, R.L. Young, A.D. Gaudet, L.K. Fonken, F.A. Champagne, J.P. CurleyDistinct immune and transcriptomic profiles in dominant versus subordinate males in mouse social hierarchiesBrain Behav Immun (2022), p. 103View PDFView articleCrossRefView in ScopusGoogle Scholar
18S.E. Border, T.J. Piefke, T.R. Funnell, R.F. Fialkowski, J. Sawecki, P.D. DijkstraSocial instability influences rank-specific patterns of oxidative stress in a cichlid fishJ Exp Biol (2021), p. 224Google Scholar
19D.J. Kostyniuk, D. Zhang, C.J. Martyniuk, K.M. Gilmour, J.A. MennigenSocial status regulates the hepatic miRNAome in rainbow trout: implications for posttranscriptional regulation of metabolic pathwaysPLoS One, 14 (2019)Google Scholar
20R. Debray, N. Snyder-Mackler, J.N. Kohn, M.E. Wilson, L.B. Barreiro, J. TungSocial affiliation predicts mitochondrial DNA copy number in female rhesus macaquesBiol Lett, 15 (2019)Google Scholar
21T. Yardeni, A.G. Cristancho, A.J. McCoy, P.M. Schaefer, M.J. McManus, E.D. Marsh, D.C. WallaceAn mtDNA mutant mouse demonstrates that mitochondrial deficiency can result in autism endophenotypesProc Natl Acad Sci U S A (2021), p. 118Google Scholar
22F. Duclot, L. Sailer, P. Koutakis, Z. Wang, M. KabbajTranscriptomic regulations underlying pair-bond formation and maintenance in the socially monogamous male and female prairie voleBiol Psychiatr (2022), p. 91Google Scholar
23∗∗A.K. Kanellopoulos, V. Mariano, M. Spinazzi, Y.J. Woo, C. McLean, U. Pech, K.W. Li, J.D. Armstrong, A. Giangrande, P. Callaerts, et al.Aralar Sequesters GABA into hyperactive mitochondria, causing social behavior deficitsCell, 180 (2020), pp. 1178-1197View in ScopusGoogle Scholar
Demonstrates that social deficits in Drosophila that are mutant for CYFIP1, a gene linked to autism and schizophrenia, are caused by GABA uptake in mitochondria, and that this process is driven by the transporter Aralar.
24F. PalmieriThe mitochondrial transporter family SLC25: identification, properties and physiopathologyMol Aspect Med, 34 (2013), pp. 465-484View PDFView articleView in ScopusGoogle Scholar
25∗∗D. Jimenez-Blasco, A. Busquets-Garcia, E. Hebert-Chatelain, R. Serrat, C. Vicente-Gutierrez, C. Ioannidou, P. Gómez-Sotres, I. Lopez-Fabuel, M. Resch-Beusher, E. Resel, et al.Glucose metabolism links astroglial mitochondria to cannabinoid effectsNature, 583 (2020)Google Scholar
Shows that low ROS in astrocytes, induced by an mtCB1 receptor mediated decrease in mitochondrial complex I function, results in decreased lactate production. This in turn, caused redox stress in neurons and, subsequently, impariments in social behaviour.
26A. Meyer-Lindenberg, C.B. Mervis, K. Faith BermanNeural mechanisms in Williams syndrome: a unique window to genetic influences on cognition and behaviourNat Rev Neurosci, 7 (2006), pp. 380-393CrossRefView in ScopusGoogle Scholar
27S.J. Sanders, A.G. Ercan-Sencicek, V. Hus, R. Luo, M.T. Murtha, D. Moreno-De-Luca, S.H. Chu, M.P. Moreau, A.R. Gupta, S.A. Thomson, et al.Multiple recurrent de novo CNVs, including duplications of the 7q11.23 Williams syndrome region, are strongly associated with autismNeuron, 70 (2011), pp. 863-885View PDFView articleView in ScopusGoogle Scholar
28A.T.N. Tebbenkamp, L. Varela, J. Choi, M.I. Paredes, A.M. Giani, J.E. Song, M. Sestan-Pesa, D. Franjic, A.M.M. Sousa, Z.W. Liu, et al.The 7q11.23 protein DNAJC30 interacts with ATP synthase and links mitochondria to brain developmentCell (2018), p. 175Google Scholar
29P.S. Ariyannur, J.R. Moffett, P. Manickam, N. Pattabiraman, P. Arun, A. Nitta, T. Nabeshima, C.N. Madhavarao, A.M.A. NamboodiriMethamphetamine-induced neuronal protein NAT8L is the NAA biosynthetic enzyme: implications for specialized acetyl coenzyme A metabolism in the CNSBrain Res, 1335 (2010), pp. 1-13View PDFView articleView in ScopusGoogle Scholar
30T.L. White, M.A. Gonsalves, R.A. Cohen, A.D. Harris, M.A. Monnig, E.G. Walsh, A.Z. Nitenson, E.C. Porges, D.G. Lamb, A.J. Woods, et al.The neurobiology of wellness: 1H-MRS correlates of agency, flexibility and neuroaffective reserves in healthy young adultsNeuroimage, 225 (2021)Google Scholar
31E. Riquin, P. Duverger, C. Cariou, M. Barth, C. Prouteau, P. Van Bogaert, D. Bonneau, A. RoyNeuropsychological and psychiatric features of children and adolescents affected with mitochondrial diseases: a systematic reviewFront Psychiatr, 11 (2020), p. 747View in ScopusGoogle Scholar
32S. Rose, D.M. Niyazov, D.A. Rossignol, M. Goldenthal, S.G. Kahler, R.E. FryeClinical and molecular characteristics of mitochondrial dysfunction in autism spectrum disorderMol Diagn Ther, 225 (2018), pp. 571-5932018, 22CrossRefView in ScopusGoogle Scholar
33J. Allen, R. Romay-Tallon, K.J. Brymer, H.J. Caruncho, L.E. KalynchukMitochondria and mood: mitochondrial dysfunction as a key player in the manifestation of depressionFront Neurosci, 12 (2018)Google Scholar
34A. Du Preez, J. Eum, I. Eiben, P. Eiben, P.A. Zunszain, C.M. Pariante, S. Thuret, C. FernandesDo different types of stress differentially alter behavioural and neurobiological outcomes associated with depression in rodent models? A systematic reviewFront Neuroendocrinol, 61 (2021), Article 100896View PDFView articleView in ScopusGoogle Scholar
35A. Papilloud, I. Guillot de Suduiraut, O. Zanoletti, J. Grosse, C. SandiPeripubertal stress increases play fighting at adolescence and modulates nucleus accumbens CB1 receptor expression and mitochondrial function in the amygdalaTransl Psychiatry, 8 (2018)Google Scholar
36∗∗L. Morató, S. Astori, I. Zalachoras, J. Rodrigues, S. Ghosal, W. Huang, I.G. De Suduiraut, J. Grosse, O. Zanoletti, L. Cao, et al.ENAMPT actions through nucleus accumbens NAD+/SIRT1 link increased adiposity with sociability deficits programmed by peripuberty stressSci Adv, 8 (2022)Google Scholar
Demonstrates that the peripubertal stress-induced increase in adiposity levels and reduction of the adipokine eNAMPT contribute to lifelong sociability deficits. ENAMPT impacts on the NAD+/SIRT1 pathway in the NAc to program sociability.
37S. Alhassen, S. Chen, L. Alhassen, A. Phan, M. Khoudari, A. De Silva, H. Barhoosh, Z. Wang, C. Parrocha, E. Shapiro, et al.Intergenerational trauma transmission is associated with brain metabotranscriptome remodeling and mitochondrial dysfunctionCommun Biol (2021), p. 4Google Scholar
38H. Amini-Khoei, A. Mohammadi-Asl, S. Amiri, M.J. Hosseini, M. Momeny, M. Hassanipour, M. Rastegar, A. Haj-Mirzaian, A.H. Mirzaian, H. Sanjarimoghaddam, et al.Oxytocin mitigated the depressive-like behaviors of maternal separation stress through modulating mitochondrial function and neuroinflammationProg Neuro-Psychopharmacol Biol Psychiatry, 76 (2017), pp. 169-178View PDFView articleView in ScopusGoogle Scholar
39S.R. Ruigrok, K. Yim, T.L. Emmerzaal, B. Geenen, N. Stöberl, J.L. den Blaauwen, M.R. Abbink, A.J. Kiliaan, E.M. van Schothorst, T. Kozicz, et al.Effects of early-life stress on peripheral and central mitochondria in male mice across agesPsychoneuroendocrinology (2021), p. 132Google Scholar
40∗∗K. Duan, Q. Gu, R.S. Petralia, Y.X. Wang, D. Panja, X. Liu, M.L. Lehmann, H. Zhu, J. Zhu, Z. LiMitophagy in the basolateral amygdala mediates increased anxiety induced by aversive social experienceNeuron, 109 (2021), pp. 3793-3809View in ScopusGoogle Scholar
Links social defeat stress susceptibility to increased mtDNA copy number mutations and mitophagy in the BLA. The anxiety-like phenotype is rescued when mitophagy is disrupted in the BLA.
41L.D. Zorova, V.A. Popkov, E.Y. Plotnikov, D.N. Silachev, I.B. Pevzner, S.S. Jankauskas, V.A. Babenko, S.D. Zorov, A.V. Balakireva, M. Juhaszova, et al.Mitochondrial membrane potentialAnal Biochem (2018), p. 552Google Scholar
42L. Guo, Z mei Jiang, R xue Sun, W. Pang, X. Zhou, M ling Du, Chen M. xiang, X. Lv, J tao WangRepeated social defeat stress inhibits development of hippocampus neurons through mitophagy and autophagyBrain Res Bull (2022), p. 182Google Scholar
43D.L. Walker, D.J. Toufexis, M. DavisRole of the bed nucleus of the stria terminalis versus the amygdala in fear, stress, and anxietyEur J Pharmacol, 463 (2003)Google Scholar
44Z. Misiewicz, S. Iurato, N. Kulesskaya, L. Salminen, L. Rodrigues, G. Maccarrone, J. Martins, D. Czamara, M.A. Laine, E. Sokolowska, et al.Multi-omics analysis identifies mitochondrial pathways associated with anxiety-related behaviorPLoS Genet, 15 (2019)Google Scholar
45N. Solanki, A. Salvi, G. Patki, S. SalimModulating oxidative stress relieves stress-induced behavioral and cognitive impairments in ratsInt J Neuropsychopharmacol, 20 (2017)Google Scholar
46B.N. Dulka, A.K. Bourdon, C.T. Clinard, M.B.K. Muvvala, S.R. Campagna, M.A. CooperMetabolomics reveals distinct neurochemical profiles associated with stress resilienceNeurobiol Stress, 7 (2017)Google Scholar
47A.P. Miranda Mendonça, L.Y. Hoppe, A. Gaviraghi, TC de Araújo-Jorge, G.M. de Oliveira, R.M. Felippe, M.F. Oliveira, V.M. da Silva FragosoHighly aggressive behavior induced by social stress is associated to reduced cytochrome c oxidase activity in mice brain cortexNeurochem Int, 126 (2019)Google Scholar
48M. Bove, A. Lama, S. Schiavone, C. Pirozzi, P. Tucci, V. Sikora, G. Trinchese, G. Corso, M.G. Morgese, L. TrabaceSocial isolation triggers oxidative status and impairs systemic and hepatic insulin sensitivity in normoglycemic ratsBiomed Pharmacother (2022), p. 149Google Scholar
49M. Sharma, S. Khan, S. Rahman, L.R. SinghThe extracellular protein, transthyretin is an oxidative stress biomarkerFront Physiol, 10 (2019)Google Scholar
50I. Perić, V. Costina, P. Gass, P. Findeisen, D. FilipovićHippocampal synaptoproteomic changes of susceptibility and resilience of male rats to chronic social isolationBrain Res Bull (2021), p. 166Google Scholar
51D. Filipović, I. Perić, V. Costina, A. Stanisavljević, P. Gass, P. FindeisenSocial isolation stress-resilient rats reveal energy shift from glycolysis to oxidative phosphorylation in hippocampal nonsynaptic mitochondriaLife Sci (2020), p. 254Google Scholar
52M.P. Cunha, D.G. Machado, G. Mancini, V. Glaser, R. de Paula Martins, A.F. de Bem, A. Latini, A.L. Dafre, A.L.S. RodriguesThe effect of voluntary wheel running on the antioxidant status is dependent on sociability conditionsPharmacol Biochem Behav (2020), p. 198Google Scholar
53L. Fan, L. Yang, X. Li, T. Teng, Y. Xiang, X. Liu, Y. Jiang, Y. Zhu, X. Zhou, P. XieProteomic and metabolomic characterization of amygdala in chronic social defeat stress ratsBehav Brain Res (2021), p. 412Google Scholar
54L. Liu, X. Zhou, Y. Zhang, J. Pu, L. Yang, S. Yuan, L. Zhao, C. Zhou, H. Zhang, P. XieHippocampal metabolic differences implicate distinctions between physical and psychological stress in four rat models of depressionTransl Psychiatry, 8 (2018)Google Scholar
55Y. Zhu, Z. Fan, Q. Zhao, J. Li, G. Cai, R. Wang, Y. Liang, N. Lu, J. Kang, D. Luo, et al.Brain-type glycogen phosphorylase is crucial for astrocytic glycogen accumulation in chronic social defeat stress-induced depression in miceFront Mol Neurosci (2022), p. 14CrossRefGoogle Scholar
56∗P.J. Hamilton, E.Y. Chen, V. Tolstikov, C.J. Peña, J.A. Picone, P. Shah, K. Panagopoulos, A.N. Strat, D.M. Walker, Z.S. Lorsch, et al.Chronic stress and antidepressant treatment alter purine metabolism and beta oxidation within mouse brain and serumSci Rep (2020), p. 10Google Scholar
Underlines that the effects of chronic social defeat are not homogenous across different brain regions. Many of the changing metabolites in the vHIP, mPFC and the NAc were involved in beta oxidation or antioxidants, or affiliated with energy metabolism.
57A. Cherix, T. Larrieu, J. Grosse, J. Rodrigues, B. McEwen, C. Nasca, R. Gruetter, C. SandiMetabolic signature in nucleus accumbens for anti-depressant-like effects of acetyl-l-carnitineElife, 9 (2020)Google Scholar
58I. Perić, V. Costina, S. Djordjević, P. Gass, P. Findeisen, D. Inta, S. Borgwardt, D. FilipovićTianeptine modulates synaptic vesicle dynamics and favors synaptic mitochondria processes in socially isolated ratsSci Rep, 11 (2021), pp. 1-15View in ScopusGoogle Scholar
59J.D. Kim, N.A. Yoon, S. Jin, S. DianoMicroglial UCP2 mediates inflammation and obesity induced by high-fat feedingCell Metabol, 30 (2019), pp. 952-962View in ScopusGoogle Scholar
60F.R. Jardim, F.T. de Rossi, M.X. Nascimento, R.G. da Silva Barros, P.A. Borges, I.C. Prescilio, M.R. de OliveiraResveratrol and brain mitochondria: a reviewMol Neurobiol, 55 (2018)Google Scholar
61F. Hollis, E.S. Mitchell, C. Canto, D. Wang, C. SandiMedium chain triglyceride diet reduces anxiety-like behaviors and enhances social competitiveness in ratsNeuropharmacology, 138 (2018)Google Scholar
62W. Wang, T. Wang, S. Bai, Z. Chen, X. Qi, P. XieDl-3-n-butylphthalide attenuates mouse behavioral deficits to chronic social defeat stress by regulating energy metabolism via AKT/CREB signaling pathwayTransl Psychiatry, 10 (2020)Google Scholar
63A.P. Toniazzo, D.M. Arcego, C. Lazzaretti, C. Mota, C.E. Schnorr, L.F. Pettenuzzo, R. Krolow, J.C. Fonseca Moreira, C. DalmazSex-dependent effect on mitochondrial and oxidative stress parameters in the hypothalamus induced by prepubertal stress and access to high fat dietNeurochem Int, 124 (2019)Google Scholar
64R.C. Froemke, L.J. YoungOxytocin, neural plasticity, and social behaviorAnnu Rev Neurosci (2021), p. 44Google Scholar
65J. Zhao, D. Qu, Z. Xi, Y. Huan, K. Zhang, C. Yu, D. Yang, J. Kang, W. Lin, S. Wu, et al.Mitochondria transplantation protects traumatic brain injury via promoting neuronal survival and astrocytic BDNFTransl Res (2021), p. 235CrossRefGoogle Scholar
66G. Javani, S. Babri, F. Farajdokht, A. Ghaffari-Nasab, G. MohaddesMitochondrial transplantation improves anxiety- and depression-like behaviors in aged stress-exposed ratsMech Ageing Dev (2022), p. 202Google Scholar
67H. Einat, P. Yuan, H.K. ManjiIncreased anxiety-like behaviors and mitochondrial dysfunction in mice with targeted mutation of the Bcl-2 gene: further support for the involvement of mitochondrial function in anxiety disordersBehav Brain Res, 165 (2005)Google Scholar
68N.S. ChandelEvolution of mitochondria as signaling organellesCell Metabol, 22 (2015), pp. 204-206View PDFView articleView in ScopusGoogle Scholar
69M. Giacomello, A. Pyakurel, C. Glytsou, L. ScorranoThe cell biology of mitochondrial membrane dynamicsNat Rev Mol Cell Biol, 214 (2020), pp. 204-2242020, 21CrossRefView in ScopusGoogle Scholar
70A. Midzak, V. PapadopoulosAdrenal mitochondria and steroidogenesis: from individual proteins to functional protein assembliesFront Endocrinol, 7 (2016)Google Scholar
71H. Chen, S.A. Detmer, A.J. Ewald, E.E. Griffin, S.E. Fraser, D.C. ChanMitofusins Mfn1 and Mfn2 coordinately regulate mitochondrial fusion and are essential for embryonic developmentJ Cell Biol (2003), p. 160Google Scholar
72D.A. Turner, D.C. AdamsonNeuronal-astrocyte metabolic interactions: understanding the transition into abnormal astrocytoma metabolismJ Neuropathol Exp Neurol, 70 (2011)Google Scholar
73D. Nolfi-Donegan, A. Braganza, S. ShivaMitochondrial electron transport chain: oxidative phosphorylation, oxidant production, and methods of measurementRedox Biol (2020), p. 37Google Scholar
74M. Sharifi-Rad, N.V. Anil Kumar, P. Zucca, E.M. Varoni, L. Dini, E. Panzarini, J. Rajkovic, P.V. Tsouh Fokou, E. Azzini, I. Peluso, et al.Lifestyle, oxidative stress, and antioxidants: back and forth in the pathophysiology of chronic diseasesFront Physiol (2020), p. 11Google Scholar
Cited by (1)
Authors contributed equally.
© 2023 The Authors. Published by Elsevier Ltd.
We use cookies to help provide and enhance our service and tailor content and ads. By continuing you agree to the use of cookies.
Copyright © 2023 Elsevier B.V. or its licensors or contributors. ScienceDirect® is a registered trademark of Elsevier B.V.
この記事が気に入ったらサポートをしてみませんか?